In vitro methylation of CpG-rich islands
Abstract
CpG islands are distinguishable from the bulk of vertebrate DNA for being unmethylated and CpG-rich. Since CpG doublets are the specific target of eukaryotic DNA methyltransferases, CpG-rich sequences might be expected to be good methyl-accepting substrates in vitro, despite their unmethylated in vivo condition. This was tested using a partially purified DNA-methyltransferase from human placenta and several cloned CpG-rich or CpG-depleted sequences. The efficiency of methylation was found to be proportional to the CpG content for CpG-depleted regions, which are representative of the bulk genome. However, methylation was much less efficient for CpG frequencies higher than 1 in 12 nucleotides, reaching only 60% of the expected level. That suggests that the close CpG spacing typical of CpG-islands somehow inhibits mammalian DNA methyltransferase. The implications of these findings on the in vivo pattern of DNA methylation are discussed.
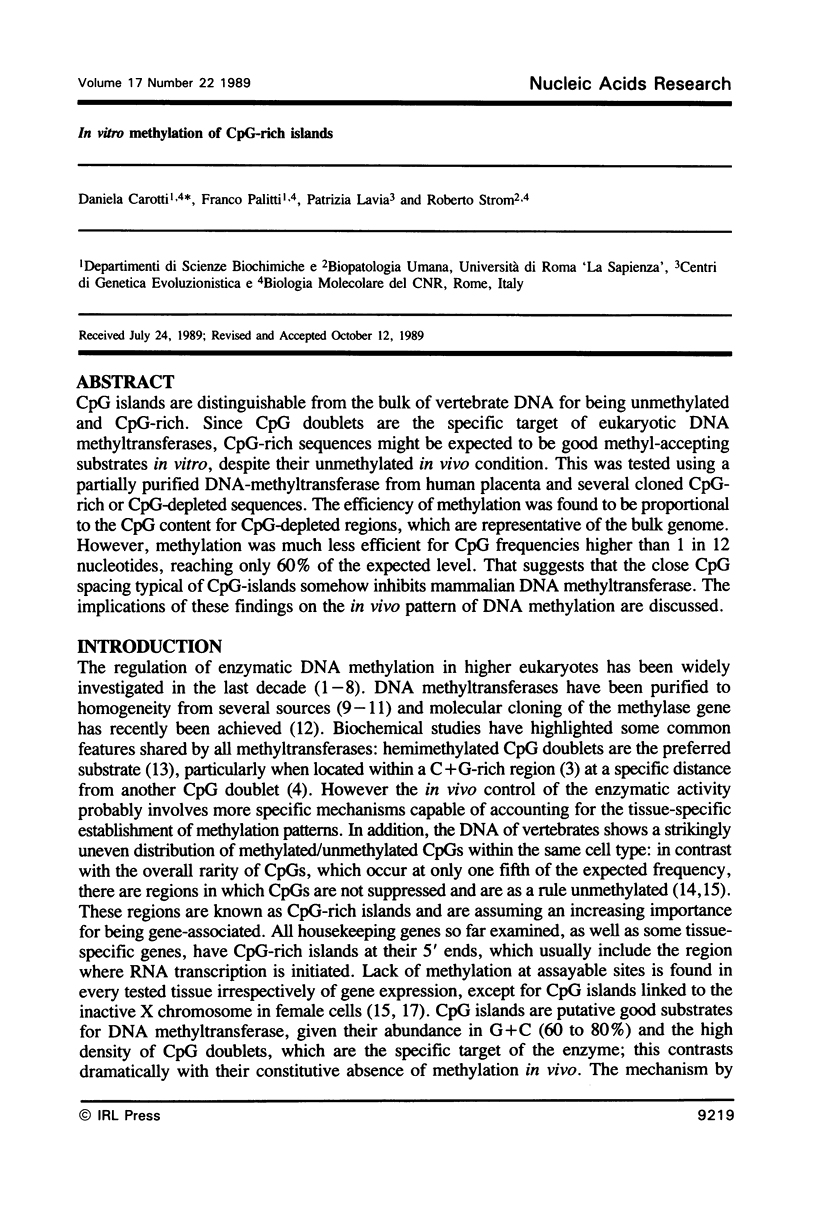
Selected References
These references are in PubMed. This may not be the complete list of references from this article.
- Antequera F., Macleod D., Bird A. P. Specific protection of methylated CpGs in mammalian nuclei. Cell. 1989 Aug 11;58(3):509–517. doi: 10.1016/0092-8674(89)90431-5. [DOI] [PubMed] [Google Scholar]
- Bestor T. H., Ingram V. M. Two DNA methyltransferases from murine erythroleukemia cells: purification, sequence specificity, and mode of interaction with DNA. Proc Natl Acad Sci U S A. 1983 Sep;80(18):5559–5563. doi: 10.1073/pnas.80.18.5559. [DOI] [PMC free article] [PubMed] [Google Scholar]
- Bestor T., Laudano A., Mattaliano R., Ingram V. Cloning and sequencing of a cDNA encoding DNA methyltransferase of mouse cells. The carboxyl-terminal domain of the mammalian enzymes is related to bacterial restriction methyltransferases. J Mol Biol. 1988 Oct 20;203(4):971–983. doi: 10.1016/0022-2836(88)90122-2. [DOI] [PubMed] [Google Scholar]
- Bestor T. Supercoiling-dependent sequence specificity of mammalian DNA methyltransferase. Nucleic Acids Res. 1987 May 11;15(9):3835–3843. doi: 10.1093/nar/15.9.3835. [DOI] [PMC free article] [PubMed] [Google Scholar]
- Bird A. P. CpG-rich islands and the function of DNA methylation. Nature. 1986 May 15;321(6067):209–213. doi: 10.1038/321209a0. [DOI] [PubMed] [Google Scholar]
- Bird A., Taggart M., Frommer M., Miller O. J., Macleod D. A fraction of the mouse genome that is derived from islands of nonmethylated, CpG-rich DNA. Cell. 1985 Jan;40(1):91–99. doi: 10.1016/0092-8674(85)90312-5. [DOI] [PubMed] [Google Scholar]
- Bolden A. H., Nalin C. M., Ward C. A., Poonian M. S., McComas W. W., Weissbach A. DNA methylation: sequences flanking C-G pairs modulate the specificity of the human DNA methylase. Nucleic Acids Res. 1985 May 24;13(10):3479–3494. doi: 10.1093/nar/13.10.3479. [DOI] [PMC free article] [PubMed] [Google Scholar]
- Bolden A. H., Nalin C. M., Ward C. A., Poonian M. S., Weissbach A. Primary DNA sequence determines sites of maintenance and de novo methylation by mammalian DNA methyltransferases. Mol Cell Biol. 1986 Apr;6(4):1135–1140. doi: 10.1128/mcb.6.4.1135. [DOI] [PMC free article] [PubMed] [Google Scholar]
- Caiafa P., Mastrantonio S., Attina M., Rispoli M., Reale A., Strom R. Do tightly-bound chromatin proteins play a role in DNA methylation? Biochem Int. 1988 Nov;17(5):863–875. [PubMed] [Google Scholar]
- Carotti D., Palitti F., Mastrantonio S., Rispoli M., Strom R., Amato A., Campagnari F., Whitehead E. P. Substrate preferences of human placental DNA methyltransferase investigated with synthetic polydeoxynucleotides. Biochim Biophys Acta. 1986 Mar 26;866(2-3):135–143. doi: 10.1016/0167-4781(86)90110-7. [DOI] [PubMed] [Google Scholar]
- Collins F. S., Weissman S. M. The molecular genetics of human hemoglobin. Prog Nucleic Acid Res Mol Biol. 1984;31:315–462. doi: 10.1016/s0079-6603(08)60382-7. [DOI] [PubMed] [Google Scholar]
- Drahovský D., Morris N. R. Mechanism of action of rat liver DNA methylase. II. Interaction with single-stranded methyl-acceptor DNA. J Mol Biol. 1971 Oct 28;61(2):343–356. doi: 10.1016/0022-2836(71)90384-6. [DOI] [PubMed] [Google Scholar]
- Gardiner-Garden M., Frommer M. CpG islands in vertebrate genomes. J Mol Biol. 1987 Jul 20;196(2):261–282. doi: 10.1016/0022-2836(87)90689-9. [DOI] [PubMed] [Google Scholar]
- Gruenbaum Y., Cedar H., Razin A. Substrate and sequence specificity of a eukaryotic DNA methylase. Nature. 1982 Feb 18;295(5850):620–622. doi: 10.1038/295620a0. [DOI] [PubMed] [Google Scholar]
- Huang L. H., Wang R., Gama-Sosa M. A., Shenoy S., Ehrlich M. A protein from human placental nuclei binds preferentially to 5-methylcytosine-rich DNA. Nature. 1984 Mar 15;308(5956):293–295. doi: 10.1038/308293a0. [DOI] [PubMed] [Google Scholar]
- Lavia P., Macleod D., Bird A. Coincident start sites for divergent transcripts at a randomly selected CpG-rich island of mouse. EMBO J. 1987 Sep;6(9):2773–2779. doi: 10.1002/j.1460-2075.1987.tb02572.x. [DOI] [PMC free article] [PubMed] [Google Scholar]
- Meehan R. R., Lewis J. D., McKay S., Kleiner E. L., Bird A. P. Identification of a mammalian protein that binds specifically to DNA containing methylated CpGs. Cell. 1989 Aug 11;58(3):499–507. doi: 10.1016/0092-8674(89)90430-3. [DOI] [PubMed] [Google Scholar]
- Palitti F., Carotti D., Grünwald S., Rispoli M., Whitehead E. P., Salerno C., Strom R., Drahovsky D. Inactivation of de novo DNA methyltransferase activity by high concentrations of double-stranded DNA. Biochim Biophys Acta. 1987 Dec 8;910(3):292–296. doi: 10.1016/0167-4781(87)90122-9. [DOI] [PubMed] [Google Scholar]
- Pfeifer G. P., Grünwald S., Palitti F., Kaul S., Boehm T. L., Hirth H. P., Drahovsky D. Purification and characterization of mammalian DNA methyltransferases by use of monoclonal antibodies. J Biol Chem. 1985 Nov 5;260(25):13787–13793. [PubMed] [Google Scholar]
- Strauss F., Varshavsky A. A protein binds to a satellite DNA repeat at three specific sites that would be brought into mutual proximity by DNA folding in the nucleosome. Cell. 1984 Jul;37(3):889–901. doi: 10.1016/0092-8674(84)90424-0. [DOI] [PubMed] [Google Scholar]
- Tomassetti A., Driever P. H., Pfeifer G. P., Drahovsky D. Isolation and characterization of proteins that stimulate the activity of mammalian DNA methyltransferase. Biochim Biophys Acta. 1988 Nov 10;951(1):201–212. doi: 10.1016/0167-4781(88)90041-3. [DOI] [PubMed] [Google Scholar]
- Toniolo D., Persico M., Alcalay M. A "housekeeping" gene on the X chromosome encodes a protein similar to ubiquitin. Proc Natl Acad Sci U S A. 1988 Feb;85(3):851–855. doi: 10.1073/pnas.85.3.851. [DOI] [PMC free article] [PubMed] [Google Scholar]
- Ward C., Bolden A., Nalin C. M., Weissbach A. In vitro methylation of the 5'-flanking regions of the mouse beta-globin gene. J Biol Chem. 1987 Aug 15;262(23):11057–11063. [PubMed] [Google Scholar]
- Wolf S. F., Migeon B. R. Clusters of CpG dinucleotides implicated by nuclease hypersensitivity as control elements of housekeeping genes. Nature. 1985 Apr 4;314(6010):467–469. doi: 10.1038/314467a0. [DOI] [PubMed] [Google Scholar]
- Zucker K. E., Riggs A. D., Smith S. S. Purification of human DNA (cytosine-5-)-methyltransferase. J Cell Biochem. 1985;29(4):337–349. doi: 10.1002/jcb.240290407. [DOI] [PubMed] [Google Scholar]