DeltaF508 CFTR protein expression in tissues from patients with cystic fibrosis - PubMed
- ️Fri Jan 01 1999
DeltaF508 CFTR protein expression in tissues from patients with cystic fibrosis
N Kälin et al. J Clin Invest. 1999.
Abstract
Heterologous expression of the cystic fibrosis transmembrane conductance regulator (CFTR) provided evidence that the major cystic fibrosis (CF) mutation DeltaF508 leads to defective protein folding in the endoplasmic reticulum, which prevents its processing and targeting to the cell surface. In this study, we investigated endogenous CFTR expression in skin biopsies and respiratory and intestinal tissue specimens from DeltaF508 homozygous and non-CF patients, using immunohistochemical and immunoblot analyses with a panel of CFTR antibodies. CFTR expression was detected at the luminal surface of reabsorptive sweat ducts and airway submucosal glands, at the apex of ciliated cells in pseudostratified respiratory epithelia and of isolated cells of the villi of duodenum and jejunum, and within intracellular compartments of intestinal goblet cells. In DeltaF508 homozygous patients, expression of the mutant protein proved to be tissue specific. Whereas DeltaF508 CFTR was undetectable in sweat glands, the expression in the respiratory and intestinal tracts could not be distinguished from the wild-type by signal intensity or localization. The tissue-specific variation of DeltaF508 CFTR expression from null to apparently normal amounts indicates that DeltaF508 CFTR maturation can be modulated and suggests that determinants other than CFTR mislocalization should play a role in DeltaF508 CF respiratory and intestinal disease.
Figures
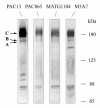
CFTR antibodies PAC13, PAC865, MATG1104, and M3A7 detect the characteristic immunoreactive CFTR bands in immunoblot analysis of T84 cells. Band C refers to mature, complex-glycosylated CFTR; band B to the immature, core-glycosylated isoform, and band A to nonglycosylated CFTR.
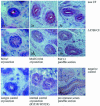
CFTR expression in sweat glands. Immunohistochemical CFTR labeling in cryosections of skin biopsies with M3A7 (1:200; 1a and 1b) and MATG1104 (1:2,500; 2a and 2b) and in paraffin sections with PAC13 (1:50; 3a and 3b), and detection with alkaline phosphatase/New Fuchsin. The different antibodies correspondingly demonstrate CFTR expression at the luminal surface of the reabsorptive duct of sweat glands in non-CF, but not ΔF508 homozygous, CF tissue specimens. Absence of the specific immunoreactive signals in different negative controls. (1c) Isotype control: M3A7 was replaced by IgG1κ; (2c) internal negative control: MATG1104 was employed for investigation of skin biopsies from patients with 2 null alleles (R553X/R553X); (3c) preimmune serum: the polyclonal CFTR antibody PAC13 was replaced by the preimmune serum of the same rabbit. ×1,453.
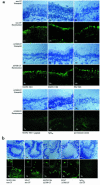
CFTR expression in the airways. (a) CFTR immunofluorescent labeling of pseudostratified areas of the surface epithelium of nasal polyps from non-CF (1–3) and ΔF508 homozygous CF patients (4–9) with MATG1061 (1a and 1b, 4a and 4b; dilution 1:200), MATG1104 (2a and 2b, 5a and 5b; dilution 1:200), and PAC865 (3a and 3b, 6a and 6b; dilution 1:200). Bottom row: Specificity is demonstrated by different negative controls. (7a and 7b) Peptide competition: 1 μg MATG1061 (1:200) was preincubated with 5 μg of CFTR peptide 503–515, Δ508; (8a and 8b) isotype control: MATG1104 was replaced by 1:200 dilution of IgG1κ; (9a and 9b) preimmune serum: the protein A affinity-purified polyclonal antibody PAC865 was replaced by the protein A affinity-purified preimmune serum (dilution 1:200) from the same rabbit. “a” panels show Nomarski view of indirect CFTR immunofluorescence in “b” panels. Fluorescence exposure times: left column = 15 sec; middle column = 15 sec; right column = 30 sec. ×2,420. (b) CFTR expression in airway submucosal gland ducts. CFTR immunolabeling was found in scattered cells of the duct of submucosal glands in non-CF (1a and 1b, 2a and 2b) and ΔF508 homozygous CF (3a and 3b, 4a and 4b) tissue specimens and is demonstrated with CFTR antibodies MATG1104 (1a and 1b, 3a and 3b; 1:200) and M3A7 (2a and 2b, 4a and 4b; 1:50). In 5a and 5b, anti-CFTR antibody was replaced by nonspecific IgG1κ (1:50). “a” panels show Nomarski view of indirect immunofluorescence in “b” panels. ×2,516. In the insets, the localization of wild-type and ΔF508 CFTR immunoreactive signals at the apex of epithelial cells is resolved at higher magnification.
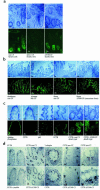
CFTR expression in the intestine. (a) CFTR immunoreactive signals of the intestine are absent in an internal negative control, a tissue specimen homozygous for 2 null alleles. CFTR immunoanalysis of a rectal biopsy from a patient homozygous for an out-of-frame deletion of exon 2 and 3 (2a and 2b) in comparison with non-CF (1a and 1b) and ΔF508 homozygous CF patients (3a and 3b), using CFTR antibody MATG1104 (1:200). Fluorescence exposure times for 1b, 2b, and 3b were 30 sec. “a” panels show Nomarski microscopy of indirect CFTR immunofluorescence in “b” panels. ×1,241. (b) General view of CFTR labeling patterns in duodenum, jejunum, and ileum of adult non-CF intestine (1–3) and newborn ileum from a CF patient suffering from meconium ileus (4b) with CFTR antibody PAC865 (1:200). Fluorescence exposure times for duodenum: 15 sec; jejunum: 30 sec; adult non-CF ileum: 30 sec; newborn ΔF508 CF ileum: 30 sec. “a” panels show Nomarski microscopy of indirect CFTR immunofluorescence in “b” panels. ×616. (c) CFTR immunolabeling of the intestine was compared with the signal pattern of marker proteins of different cellular membranes. CFTR expression in isolated intestinal cells of the villi in duodenum and jejunum (2a and 2b, arrow; MATG1104, 1:200) appears apical when compared with a marker of the luminal cell membrane of intestinal villi (1a and 1b; alkaline phosphatase). Similar immunoreactive labeling patterns of CFTR (4a and 4b; PAC13, 1:200) and a marker protein for the ER (3a and 3b; p63) in intermediate crypts of jejunum, labeling of both proteins predominantly within mucus-secreting cells. Identical CFTR immunoreactivity in deep crypts of the ileum of non-CF (5a and 5b; PAC865, 1:200) and ΔF508 homozygous CF patients (6a and 6b; PAC865, 1:200). Fluorescence exposure times: 1b = 20 sec; 2b = 60 sec; 3b = 60 sec; 4b = 30 sec; 5b = 15 sec; 6b = 15 sec. “a” panels show Nomarski microscopy of indirect CFTR immunofluorescence in “b” panels. ×2,467. (d) CFTR immunolabeling in paraffin-embedded intestinal tissue specimens with PAC13 (1:50) and signal detection with horseradish peroxidase and DAB/nickel chloride as chromogen substrate. CFTR immunolabeling within intestinal goblet cells (1a) was forced out by peptide competition with CFTR immunogen (CFTR 1468–1480) (1b). Comparison of CFTR immunolabeling of non-CF (2a) and ΔF508 homozygous CF ileum (2b) in general view. CFTR immunolocalization within goblet cells in a high-magnification view demonstrates that CFTR signals are intracellular (3b) and overlap with the spatial distribution of the trans-Golgi network, as indicated by γ-adaptin immunolabeling (3a). CFTR labeling within goblet cells is indistinguishable in subcellular localization and intensity in non-CF (4a and 5a) and ΔF508 homozygous CF tissues (4b and 5b). 1a, 1b, 2a, 2b: ×616. 3a, 3b, 4a, 4b, 5a, 5b: ×6,168.
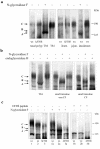
Immunoblot analysis of CFTR expression in nasal polyps and the intestine of non-CF and ΔF508 homozygous CF patients. Discrimination of CFTR glycoforms by deglycosylation (generation of unglycosylated band A) with N-glycosidase F and endoglycosidase H; the latter reacts with core-glycosylated CFTR (band B) but not with mature CFTR (band C). Deglycosylations were for 3 hours at 37°C in the presence of protease inhibitors. (a) The major CFTR immunoreactive bands of non-CF and ΔF508 homozygous CF nasal polyps and intestinal tissue specimens were indistinguishable from complex-glycosylated band C of T84 cells. In the intestine, CFTR expression was highest in membrane preparations from duodenum and decreased in tissue specimens from jejunum and ileum. Immunodetection was performed with MATG1104 (1:500). (b) Complex modification of the major CFTR immunoreactive signal of ΔF508 homozygous CF intestine (right) is demonstrated by sensitivity to N-glycosidase F and resistance to endoglycosidase H, as is the case for mature CFTR of control non-CF intestine (middle) and T84 cells (left). Immunodetection with M3A7 (1:500). (c) Specificity of the immunoreactive bands from intestinal tissue specimens for CFTR is demonstrated by the characteristic mobility shifts in deglycosylation assays with N-glycosidase F and endoglycosidase H and by peptide competition for antibody binding with the CFTR immunogen. (1) From adult non-CF duodenum; (2) from jejunum of the same patient; (3) from adult ileum of a ΔF508 homozygous CF patient. “a” lanes show deglycosylation of the membrane preparations with N-glycosidase F. “b” lanes show peptide competition with the CFTR immunogen (1 μg MATG1104 preincubated at 20°C with 5 μg CFTR peptide 722–734). Immunodetection with MATG1104 (1:500).
Comment in
-
What happens to deltaF508 in vivo?
Drumm M. Drumm M. J Clin Invest. 1999 May 15;103(10):1369-70. doi: 10.1172/JCI7119. J Clin Invest. 1999. PMID: 10330418 Free PMC article. No abstract available.
Similar articles
-
Oglesby IK, Chotirmall SH, McElvaney NG, Greene CM. Oglesby IK, et al. J Immunol. 2013 Apr 1;190(7):3354-62. doi: 10.4049/jimmunol.1202960. Epub 2013 Feb 22. J Immunol. 2013. PMID: 23436935
-
The DeltaF508 mutation results in loss of CFTR function and mature protein in native human colon.
Mall M, Kreda SM, Mengos A, Jensen TJ, Hirtz S, Seydewitz HH, Yankaskas J, Kunzelmann K, Riordan JR, Boucher RC. Mall M, et al. Gastroenterology. 2004 Jan;126(1):32-41. doi: 10.1053/j.gastro.2003.10.049. Gastroenterology. 2004. PMID: 14699484
-
Kreda SM, Mall M, Mengos A, Rochelle L, Yankaskas J, Riordan JR, Boucher RC. Kreda SM, et al. Mol Biol Cell. 2005 May;16(5):2154-67. doi: 10.1091/mbc.e04-11-1010. Epub 2005 Feb 16. Mol Biol Cell. 2005. PMID: 15716351 Free PMC article.
-
Reddy MM, Quinton PM. Reddy MM, et al. JOP. 2001 Jul;2(4 Suppl):212-8. JOP. 2001. PMID: 11875262 Review.
-
Belcher CN, Vij N. Belcher CN, et al. Curr Mol Med. 2010 Feb;10(1):82-94. doi: 10.2174/156652410791065408. Curr Mol Med. 2010. PMID: 20205681 Free PMC article. Review.
Cited by
-
Liu J, Walker NM, Ootani A, Strubberg AM, Clarke LL. Liu J, et al. J Clin Invest. 2015 Mar 2;125(3):1056-68. doi: 10.1172/JCI73193. Epub 2015 Feb 2. J Clin Invest. 2015. PMID: 25642775 Free PMC article.
-
Single-Cell RNA Sequencing Reveals New Basic and Translational Insights in the Cystic Fibrosis Lung.
Januska MN, Walsh MJ. Januska MN, et al. Am J Respir Cell Mol Biol. 2023 Feb;68(2):131-139. doi: 10.1165/rcmb.2022-0038TR. Am J Respir Cell Mol Biol. 2023. PMID: 36194688 Free PMC article. Review.
-
CFTR Modulators: Does One Dose Fit All?
van der Meer R, Wilms EB, Heijerman HGM. van der Meer R, et al. J Pers Med. 2021 May 24;11(6):458. doi: 10.3390/jpm11060458. J Pers Med. 2021. PMID: 34073663 Free PMC article. Review.
-
Yeh JT, Hwang TC. Yeh JT, et al. J Physiol. 2020 Feb;598(3):517-541. doi: 10.1113/JP278418. Epub 2019 Nov 2. J Physiol. 2020. PMID: 31585024 Free PMC article.
-
Rescuing protein conformation: prospects for pharmacological therapy in cystic fibrosis.
Gelman MS, Kopito RR. Gelman MS, et al. J Clin Invest. 2002 Dec;110(11):1591-7. doi: 10.1172/JCI16786. J Clin Invest. 2002. PMID: 12464661 Free PMC article. Review. No abstract available.
References
-
- Welsh, M.J., Tsui, L.-C., Boat, T.F., and Beaudet, A.L. 1995. Cystic fibrosis. In The metabolic and molecular bases of inherited disease. 7th edition. C.R.Scriver, A.L. Beaudet, W.S. Sly, and D. Valle, editors. McGraw-Hill. New York, NY. 3799–3876.
-
- Cheng SH, et al. Defective intracellular transport and processing of CFTR is the molecular basis of most cystic fibrosis. Cell. 1990;63:827–834. - PubMed
-
- Zhang F, Kartner N, Lukacs GL. Limited proteolysis as a probe for arrested conformational maturation of ΔF508 CFTR. Nat Struct Biol. 1998;5:180–183. - PubMed
-
- Pind S, Riordan JR, Williams DB. Participation of the endoplasmic reticulum chaperone calnexin (p88, IP90) in the biogenesis of the cystic fibrosis transmembrane conductance regulator. J Biol Chem. 1994;269:12784–12788. - PubMed
Publication types
MeSH terms
Substances
LinkOut - more resources
Full Text Sources
Other Literature Sources
Medical
Miscellaneous