The actions of ether, alcohol and alkane general anaesthetics on GABAA and glycine receptors and the effects of TM2 and TM3 mutations - PubMed
Comparative Study
The actions of ether, alcohol and alkane general anaesthetics on GABAA and glycine receptors and the effects of TM2 and TM3 mutations
M D Krasowski et al. Br J Pharmacol. 2000 Feb.
Abstract
The actions of 13 general anaesthetics (diethyl ether, enflurane, isoflurane, methoxyflurane, sevoflurane, chloral hydrate, trifluoroethanol, tribromoethanol, tert-butanol, chloretone, brometone, trichloroethylene, and alpha-chloralose) were studied on agonist-activated Cl(-) currents at human GABA(A) alpha(2)beta(1), glycine alpha(1), and GABA(C) rho(1) receptors expressed in human embryonic kidney 293 cells. All 13 anaesthetics enhanced responses to submaximal (EC(20)) concentrations of agonist at GABA(A) and glycine receptors, except alpha-chloralose, which did not enhance responses at the glycine alpha(1) receptor. None of the anaesthetics studied potentiated GABA responses at the GABA(C) rho(1) receptor. Potentiation of submaximal agonist currents by the anaesthetics was studied at GABA(A) and glycine receptors harbouring mutations in putative transmembrane domains 2 and 3 within GABA(A) alpha(2), beta(1), or glycine alpha(1) receptor subunits: GABA(A) alpha(2)(S270I)beta(1), alpha(2)(A291W)beta(1), alpha(2)beta(1)(S265I), and alpha(2)beta(1)(M286W); glycine alpha(1)(S267I) and alpha(1)(A288W). For all anaesthetics studied except alpha-chloralose, at least one of the mutations above abolished drug potentiation of agonist responses at GABA(A) and glycine receptors. alpha-Chloralose produced efficacious direct activation of the GABA(A) alpha(2)beta(1) receptor (a 'GABA-mimetic' effect). The other 12 anaesthetics produced minimal or no direct activation of GABA(A) and glycine receptors. A non-anaesthetic isomer of alpha-chloralose, beta-chloralose, was inactive at GABA(A) and glycine receptors and did not antagonize the actions of alpha-chloralose at GABA(A) receptors. The implications of these findings for the molecular mechanisms of action of general anaesthetics at GABA(A) and glycine receptors are discussed.
Figures
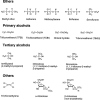
Chemical structures of the anaesthetic compounds investigated in this study.
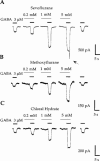
Potentiation of current responses to EC20 concentrations of GABA at wild-type human GABAA α2β1 receptors by sevoflurane (A), methoxyflurane (B), and chloral hydrate (C). Note the ‘rebound' current (arrow) following washout of 5 m
Msevoflurane. Both sevoflurane and methoxyflurane directly activate the GABAA α2β1 receptor, particulary noticeable during the pre-application of 5 m
Msevoflurane or methoxyflurane. Traces shown are individual recordings from HEK 293 cells transfected with cDNAs encoding the GABAA α2 and β1 receptor subunits.
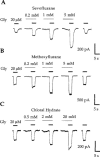
Potentiation of current responses to EC20 concentrations of glycine (Gly) at wild-type human glycine α1 receptors by sevoflurane (A), methoxyflurane (B), and chloral hydrate (C). Sevoflurane (5 m
M) produces slight direct activation of the glycine α1 receptor. Traces shown are individual recordings from HEK 293 cells transfected with cDNA encoding the glycine α1 receptor subunit.
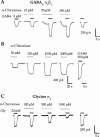
α-Chloralose produces potentiation of GABA responses and direct activation at the GABAA α2β1 receptor, yet inhibits submaximal glycine responses at the glycine α1 receptor. (A) α-Chloralose potentiates current responses to EC20 concentrations of GABA at the GABAA α2β1 receptor. Direct activation is evident during the pre-application of 50 and 200 μ
Mα-chloralose. (B) α-Chloralose directly activates the GABAA α2β1 receptor. The first four traces are for α-chloralose applied in the absence of any GABA in the perfusion system. The response to a maximal concentration of GABA (500 μ
M) is shown for comparison. Note the slow time course of the α-chloralose-mediated currents. (C) α-Chloralose, at concentrations greater than 100 μ
M, inhibits current responses to EC20 concentrations of glycine (Gly) at the glycine α1 receptor. Traces shown are individual recordings from HEK 293 cells transfected with cDNAs encoding the GABAA α2 and β1 receptor subunits (A,B) or the glycine α1 receptor subunit (C).
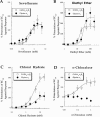
Pooled concentration-response relationships for (A) sevoflurane, (B) diethyl ether, (C) chloral hydrate, and (D) α-chloralose modulation of currents in response to submaximal (EC20) concentrations of agonist at wild-type GABAA α2β1 and glycine α1 receptors. Note the lack of effect of α-chloralose at the glycine α1 receptor at concentrations ⩽0.1 m
Mwith inhibition of glycine responses evident at 0.2, 0.5, and 1 m
M. See Table 1 for EC50, Hill slope, Emax, and ncells values.
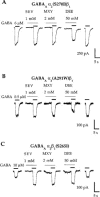
Mutations in TM2 or TM3 of the GABAA α2 subunit abolish potentiation of responses to EC20 concentrations of GABA by the ether anaesthetics sevoflurane (SEV), methoxyflurane (MXY), and diethyl ether (DEE). (A,B) Sevoflurane (1 m
M) and methoxyflurane (2 m
M) fail to potentiate submaximal GABA currents, while diethyl ether (50 m
M) inhibits GABA responses at GABAA α2(S270I)β1 and GABAA α2(A291W)β1 receptors. (C) In contrast, sevoflurane, methoxyflurane, and diethyl ether potentiate submaximal GABA responses at the GABAA α2β1(S265I) receptor. Note that the concentrations of GABA required to produce 20% of a maximal response (i.e., the EC20 concentration) are different for each of the receptors depicted in (A), (B), and (C). Some of the receptors containing mutated subunits have apparent affinities for GABA that differ from that at the wild-type GABAA α2β1 receptor. Traces shown are individual recordings from HEK 293 cells transfected with cDNAs encoding the indicated receptor subunit combinations.
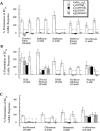
Summary of the effects of mutations in TM2 or TM3 of GABAA α2 or β1 receptor subunits on potentiation of GABA responses by general anaesthetics. The general anaesthetics are sorted into (A) ethers, (B) primary alcohols along with trichloroethylene and propofol, and (C) tertiary alcohols and α-chloralose. The ordinate depicts percentage change of a control response to an EC20 concentration of GABA by co-application with anaesthetic (where 0%=no effect). The amount of potentiation produced by an anaesthetic at a given mutated receptor was compared to the corresponding potentiation produced at the wild-type GABAA α2β1 receptor. *, **, or *** indicates that the amount of potentiation at the mutated receptor was different from that at the wild-type GABAA α2β1 receptor with a significance value of P<0.05, P<0.01, or P<0.005, respectively. Data for isoflurane, propofol (Krasowski et al., 1998b) and TCEt (Krasowski et al., 1998a) have been previously published.
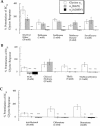
Summary of the effects of mutations in TM2 or TM3 of the glycine α1 receptor subunit on potentiation of submaximal glycine currents by (A) ethers, (B) primary alcohols along with the alkane trichloroethylene, and (C) tertiary alcohols. The ordinate depicts percentage change of a control response to an EC20 concentration of glycine by co-application with anaesthetic. The amount of potentiation produced by an anaesthetic at a given mutated receptor was compared to the corresponding potentiation produced at the wild-type glycine α1 receptor. *, **, or *** indicates that the amount of potentiation at the mutant receptor was different from that at the wild-type glycine α1 receptor with a significance value of P<0.05, P<0.01, or P<0.005, respectively. Data for TCEt has been previously published (Krasowski et al., 1998a).
Similar articles
-
Pistis M, Belelli D, Peters JA, Lambert JJ. Pistis M, et al. Br J Pharmacol. 1997 Dec;122(8):1707-19. doi: 10.1038/sj.bjp.0701563. Br J Pharmacol. 1997. PMID: 9422818 Free PMC article.
-
Trichloroethanol modulation of recombinant GABAA, glycine and GABA rho 1 receptors.
Krasowski MD, Finn SE, Ye Q, Harrison NL. Krasowski MD, et al. J Pharmacol Exp Ther. 1998 Mar;284(3):934-42. J Pharmacol Exp Ther. 1998. PMID: 9495852
-
Nishikawa K, Harrison NL. Nishikawa K, et al. Anesthesiology. 2003 Sep;99(3):678-84. doi: 10.1097/00000542-200309000-00024. Anesthesiology. 2003. PMID: 12960553
-
Mechanism of action of general anaesthetics--new information from molecular pharmacology.
Thompson SA, Wafford K. Thompson SA, et al. Curr Opin Pharmacol. 2001 Feb;1(1):78-83. doi: 10.1016/s1471-4892(01)00013-3. Curr Opin Pharmacol. 2001. PMID: 11712540 Review.
-
General anaesthetics and their effects on GABA(A) receptor desensitization.
Orser BA, McAdam LC, Roder S, MacDonald JF. Orser BA, et al. Toxicol Lett. 1998 Nov 23;100-101:217-24. doi: 10.1016/s0378-4274(98)00188-x. Toxicol Lett. 1998. PMID: 10049145 Review.
Cited by
-
Windahl U, Tevell Åberg A, Kryuchkov F, Lundgren S, Tegner C, Dreimanis K, Koivisto S, Simola O, Sandvik M, Bernhoft A. Windahl U, et al. BMC Vet Res. 2022 Sep 5;18(1):334. doi: 10.1186/s12917-022-03370-w. BMC Vet Res. 2022. PMID: 36064401 Free PMC article.
-
Nitrous oxide plus isoflurane induces apoptosis and increases beta-amyloid protein levels.
Zhen Y, Dong Y, Wu X, Xu Z, Lu Y, Zhang Y, Norton D, Tian M, Li S, Xie Z. Zhen Y, et al. Anesthesiology. 2009 Oct;111(4):741-52. doi: 10.1097/ALN.0b013e3181b27fd4. Anesthesiology. 2009. PMID: 19741497 Free PMC article.
-
New insights into the molecular mechanisms of general anaesthetics.
Chau PL. Chau PL. Br J Pharmacol. 2010 Sep;161(2):288-307. doi: 10.1111/j.1476-5381.2010.00891.x. Br J Pharmacol. 2010. PMID: 20735416 Free PMC article. Review.
-
Luethy A, Boghosian JD, Srikantha R, Cotten JF. Luethy A, et al. Mol Pharmacol. 2017 Jun;91(6):620-629. doi: 10.1124/mol.117.108290. Epub 2017 Mar 21. Mol Pharmacol. 2017. PMID: 28325748 Free PMC article.
-
Salous AK, Ren H, Lamb KA, Hu XQ, Lipsky RH, Peoples RW. Salous AK, et al. Br J Pharmacol. 2009 Nov;158(5):1395-404. doi: 10.1111/j.1476-5381.2009.00397.x. Epub 2009 Sep 25. Br J Pharmacol. 2009. PMID: 19788495 Free PMC article.
References
-
- ALLOTT P.R., STEWARD A., FLOOK V., MAPLESON W.W. Variation with temperature of the solubilities of inhaled anaesthetics in water, oil and biological media. Br. J. Anaesth. 1973;45:294–300. - PubMed
-
- BANKS M.I., PEARCE R.A. Dual actions of volatile anesthetics on GABAA IPSCs: dissociation of blocking and prolonging effects. Anesthesiol. 1999;90:120–134. - PubMed
-
- BARNARD E.A., SKOLNICK P., OLSEN R.W., MOHLER H., SIEGHART W., BIGGIO G., BRAESTRUP C., BATESON A.N., LANGER S.Z. International union of pharmacology. XV. Subtypes of γ-aminobutyric acidA receptors: classification on the basis of subunit structure and receptor function. Pharmacol. Rev. 1998;50:291–313. - PubMed
Publication types
MeSH terms
Substances
Grants and funding
LinkOut - more resources
Full Text Sources
Other Literature Sources