Integrin dynamics and matrix assembly: tensin-dependent translocation of alpha(5)beta(1) integrins promotes early fibronectin fibrillogenesis - PubMed
- ️Sat Jan 01 2000
Integrin dynamics and matrix assembly: tensin-dependent translocation of alpha(5)beta(1) integrins promotes early fibronectin fibrillogenesis
R Pankov et al. J Cell Biol. 2000.
Abstract
Fibronectin matrix assembly is a multistep, integrin-dependent process. To investigate the role of integrin dynamics in fibronectin fibrillogenesis, we developed an antibody-chasing technique for simultaneous tracking of two integrin populations by different antibodies. We established that whereas the vitronectin receptor alpha(v)beta(3) remains within focal contacts, the fibronectin receptor alpha(5)beta(1) translocates from focal contacts into and along extracellular matrix (ECM) contacts. This escalator-like translocation occurs relative to the focal contacts at 6.5 +/- 0.7 microm/h and is independent of cell migration. It is induced by ligation of alpha(5)beta(1) integrins and depends on interactions with a functional actin cytoskeleton and vitronectin receptor ligation. During cell spreading, translocation of ligand-occupied alpha(5)beta(1) integrins away from focal contacts and along bundles of actin filaments generates ECM contacts. Tensin is a primary cytoskeletal component of these ECM contacts, and a novel dominant-negative inhibitor of tensin blocked ECM contact formation, integrin translocation, and fibronectin fibrillogenesis without affecting focal contacts. We propose that translocating alpha(5)beta(1) integrins induce initial fibronectin fibrillogenesis by transmitting cytoskeleton-generated tension to extracellular fibronectin molecules. Blocking this integrin translocation by a variety of treatments prevents the formation of ECM contacts and fibronectin fibrillogenesis. These studies identify a localized, directional, integrin translocation mechanism for matrix assembly.
Figures
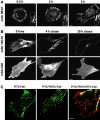
Cell surface dynamics of β1 integrins examined by conventional immunofluorescence (A) and an antibody-chasing technique (B and C). (A) HFF were allowed to spread on coverslips in complete medium for the indicated periods of time, then fixed and stained with anti–β1 integrin antibody (mAb 9EG7). (B) Overnight cultures of HFF in complete medium were labeled in vivo for 30 min with mAb 12G10 or mAb K20. Unbound antibodies were rinsed away, and labeled cells were fixed at 0 time, or chased for 4 or 30 h, then fixed and stained with secondary antibody without permeabilization. Cell borders were traced from the corresponding phase-contrast images. (C) HFF were labeled in succession for 30 min each with FITC-conjugated mAb 12G10 Fab′ fragments (FITC-Fab′), followed by rhodamine-conjugated 12G10 Fab′ (FITC/TRITC-Fab′), then again with FITC-12G10 Fab′ (FITC/TRITC/FITC-Fab′). The last two panels are merged digital FITC and rhodamine images. Bars, 10 μm.
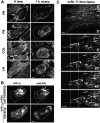
Identification of the translocating integrin heterodimer. (A) HFF were cultured overnight on VN-coated coverslips in FN-free medium with 25 μg/ml cycloheximide, and then labeled for 30 min in vivo with anti–β1 integrin antibody mAb 12G10. Cells were fixed either without chasing (0 time) or after a 1-h chase. Antibody labeling and chasing were performed in the presence of 50 μg/ml of the following ECM proteins: VN, FN, collagen I (COL), or laminin 1 (LN). Note that only FN was able to induce translocation of the β1 integrin signal out of FC. Bar, 10 μm. (B) HFF were cultured in complete medium containing 50 μg/ml of anti-α5 antibodies mAb 11 (noninhibitory) or mAb 16 (function-blocking). The cells were assayed by a 1-h chase of β1 integrins with mAb 12G10 (anti-β1), fixed, and double-stained with anti-FN. Cell contours were traced from corresponding phase-contrast images. Bar, 10 μm. (C) HFF were cultured overnight in complete medium on glass coverslips, then labeled with 10 μg/ml Alexa 488–tagged anti–α5 integrin antibody mAb 11 for 20 min. Samples were rinsed and analyzed by time-lapse microscopy at 20-min intervals as described in Materials and Methods. The top panel shows the first image, and the dashed line outlines the region shown enlarged in the lower four panels. Arrows indicate α5 integrins extending in a fibrillar structure from FC. Asterisks indicate a nonmotile aggregate relative to the fixed fiduciary lines. Bar, 5 μm.
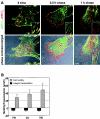
Comparisons of β1 and β3 integrin dynamics. (A) Overnight cultures of HFF on glass coverslips in complete medium were double-labeled in vivo with anti-β1 (rat mAb 9EG7) and anti-β3 (mouse mAb AP3) antibodies for 30 min. After rinsing, the cells were fixed without chasing (0 time) or after chasing for 0.5 or 1 h. Fixed, nonpermeabilized cells were stained with a mixture of FITC-conjugated anti–rat and CY3-conjugated anti–mouse goat IgG for visualization of β1 and β3 integrins, respectively. FITC and CY3 immunofluorescence images were overlaid and merged with the corresponding phase-contrast images. Bar, 10 μm. (B) HFF were plated on glass coverslips (GL), or coverslips coated with 10 μg/ml FN or VN and cultured for 16 h in complete medium. Cell motility was recorded by time-lapse video microscopy for 6 h. Corresponding immunofluorescence samples were prepared as described in A and used to measure the rate of separation between β1 and β3 integrin signals as described in Materials and Methods. Data were pooled from three independent experiments, each one measuring at least 10 cells. Error bars indicate SD.
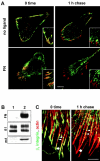
Dependence of integrin translocation on receptor ligation and interactions with actin cytoskeleton. (A) HFF were cultured as described in Fig. 2 A and then double-labeled in vivo for 30 min with anti-β1 (mAb 9EG7) and anti–β3 integrin (mAb AP3) antibodies (0 time), or chased for 1 h. Labeling and chasing were performed either with no ligand or in the presence of 100 μg/ml human plasma FN. Insets show enlargements; the bottom right panel inset was from an additional sample chased for only 30 min. Bar, 10 μm. (B) Activated β1 integrins were immunoprecipitated with mAb 9EG7 from HFF grown overnight in FN-free medium with 10 μg/ml cycloheximide without (lane 1) or with 100 μg/ml human plasma FN (lane 2). Immunoprecipitates were immunoblotted for coprecipitating FN, β1 integrin (β1), and actin (act). (C) Nontreated HFF grown in complete medium were labeled in vivo for 30 min with mAb 9EG7, fixed, and double-stained with FITC-conjugated anti–rat secondary antibody and rhodamine-phalloidin to visualize F-actin (0 time), or chased for 1 h before fixation and staining with the same mixture. Arrows indicate the position of FC, and arrowheads indicate integrin complexes along stress fibers. Bar, 5 μm.
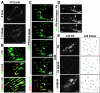
Cell surface dynamics of FN. (A) HFF were treated with cycloheximide as described in Fig. 2 A and incubated in vivo for 30 min with 10 μg/ml FITC-conjugated FN. The FN label was fixed at 0 time or chased for 1 h before fixation. (B) Cycloheximide-treated HFF were labeled for 30 min in succession with 10 μg/ml Alexa 488–conjugated FN (488-FN), followed by Alexa 594–conjugated FN (488/594-FN), and finally Alexa 488–FN again (488/594/488-FN). The last two panels represent digital overlays of images obtained from the green and red channels. (C and D) HFF were cultured overnight in FN-free medium supplemented with 10 μg/ml cycloheximide, then incubated with 10 μg/ml Alexa 488–conjugated FN for 30 min. Video recording was performed in FN-free medium (C, 488-FN time-lapse) or medium containing 100 μg/ml unlabeled FN (D, 488-FN time-lapse/FN). The fibrils in D extended ∼4 μm in 40 min. At the end of the recording, samples were fixed and stained with anti–β1 integrin antibody (mAb 9EG7) and CY3-conjugated secondary antibody, and the recorded cells were examined again to determine the position of β1 integrins and labeled FN. Note the complete overlap between β1 integrin (red) and translocated labeled FN (green), resulting in yellow staining of ECM contacts in merged images, whereas FC are only β1 integrin–positive (C, bottom panel). Fiduciary lines indicate initial positions, arrows indicate tips of translocating fibril, and asterisks mark nonmotile FN aggregates. (E) Cycloheximide-treated HFF in FN-free medium were incubated with (FN) or without (no ligand) 100 μg/ml human plasma FN and recorded by time-lapse video microscopy for 6 h. Untreated cells grown in complete medium were used as controls. Representative examples of cell movements tracked at 30-min intervals over a span of 3 h are shown (cell tracks in right panels). At the end of the recording, the cells were fixed and stained with FITC-conjugated anti-FN antibodies (anti-FN). Cell borders were traced from corresponding phase-contrast images. Bars: (A and E) 10 μm; (B, C and D) 5 μm.
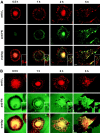
Distribution of β1 integrins and FN during cell spreading. HFF were allowed to spread in complete medium on coverslips coated with 10 μg/ml VN (A) or FN (B) for the indicated times. Cells were fixed and double-stained for β1 integrins with mAb 9EG7 (anti-β1) and FITC-conjugated anti–human FN antibody (anti-FN). Immunofluorescence images of each cell were merged by image processing. Bars, 10 μm.
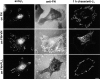
Ligation of VN receptor is necessary for α5β1 integrin translocation and FN fibrillogenesis. HFF were allowed to spread for 4 h on coverslips coated with 10 μg/ml FN, a mixture of FN and VN (5 μg/ml each), or a mixture of FN and poly-
l-lysine (5 μg/ml) in serum-free medium. Cells were fixed, permeabilized, and double-stained with mAb 9EG7 (anti-β1) and anti-FN antibodies, or β1 integrins were labeled in vivo for 30 min and chased for 1 h (1 h chase/anti-β1). Bar, 10 μm.
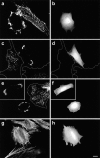
Experimental suppression of integrin translocation and FN fibrillogenesis by the tensin domain 659–762. HFF were transfected with the GFP-tagged actin homology 2 fragment of tensin and used after 36 h for in vivo labeling (a and b) and 1 h chasing of β1 integrins (c and d). Additional transfected samples were stained with anti-FN antibodies (e and f) and TRITC-phalloidin (g and h). Overexpressing cells were identified by GFP fluorescence (b, d, f, and h). Cell borders were traced from corresponding phase-contrast images. Bar, 10 μm.
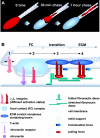
Models of integrin dynamics and FN matrix assembly. (A) Schematic representation of the translocation of labeled α5β1 integrins (red) out of FC (blue) and along actin filaments (gray) as characterized in this study. (B) Model of early FN fibrillogenesis driven by integrin translocation. (1) Nonactivated integrins are diffusely distributed on the cell surface. (2) Within FC, some α5β1 integrins can be activated even in the absence of FN (see text for details). (3) FN binding to α5β1 integrins induces formation of homogeneous clusters of this integrin; the β1 integrin cytoplasmic domains organize tensin-containing ECM contact complexes capable of translocating along actin filaments. (4) Moving ECM contact complexes pull α5β1 integrin clusters out of FC into new fibrillar ECM contacts. We propose that translocating integrins in turn pull and stretch bound FN molecules, which unfold and expose cryptic self-association sites necessary for subsequent FN polymerization. Note that components are drawn schematically and not to scale.
Similar articles
-
N-cadherin cell-cell adhesion complexes are regulated by fibronectin matrix assembly.
Lefort CT, Wojciechowski K, Hocking DC. Lefort CT, et al. J Biol Chem. 2011 Jan 28;286(4):3149-60. doi: 10.1074/jbc.M110.115733. Epub 2010 Nov 17. J Biol Chem. 2011. PMID: 21084302 Free PMC article.
-
Regulation of integrin-mediated adhesion at focal contacts by cyclic AMP.
Glass WF 2nd, Kreisberg JI. Glass WF 2nd, et al. J Cell Physiol. 1993 Nov;157(2):296-306. doi: 10.1002/jcp.1041570212. J Cell Physiol. 1993. PMID: 7693723
-
Selective regulation of integrin--cytoskeleton interactions by the tyrosine kinase Src.
Felsenfeld DP, Schwartzberg PL, Venegas A, Tse R, Sheetz MP. Felsenfeld DP, et al. Nat Cell Biol. 1999 Aug;1(4):200-6. doi: 10.1038/12021. Nat Cell Biol. 1999. PMID: 10559917
-
Fibronectin fibrillogenesis, a cell-mediated matrix assembly process.
Mao Y, Schwarzbauer JE. Mao Y, et al. Matrix Biol. 2005 Sep;24(6):389-99. doi: 10.1016/j.matbio.2005.06.008. Matrix Biol. 2005. PMID: 16061370 Review.
-
Integrins in cell adhesion and signaling.
Akiyama SK. Akiyama SK. Hum Cell. 1996 Sep;9(3):181-6. Hum Cell. 1996. PMID: 9183647 Review.
Cited by
-
Summerbell ER, Mouw JK, Bell JSK, Knippler CM, Pedro B, Arnst JL, Khatib TO, Commander R, Barwick BG, Konen J, Dwivedi B, Seby S, Kowalski J, Vertino PM, Marcus AI. Summerbell ER, et al. Sci Adv. 2020 Jul 24;6(30):eaaz6197. doi: 10.1126/sciadv.aaz6197. eCollection 2020 Jul. Sci Adv. 2020. PMID: 32832657 Free PMC article.
-
Mechanosensitive Regulation of Fibrosis.
Yang S, Plotnikov SV. Yang S, et al. Cells. 2021 Apr 23;10(5):994. doi: 10.3390/cells10050994. Cells. 2021. PMID: 33922651 Free PMC article. Review.
-
Papp S, Fadel MP, Michalak M, Opas M. Papp S, et al. Mol Cell Biochem. 2008 Jan;307(1-2):237-48. doi: 10.1007/s11010-007-9602-7. Epub 2007 Oct 2. Mol Cell Biochem. 2008. PMID: 17909946
-
Datta A, Shi Q, Boettiger DE. Datta A, et al. Mol Cell Biol. 2001 Nov;21(21):7295-306. doi: 10.1128/MCB.21.21.7295-7306.2001. Mol Cell Biol. 2001. PMID: 11585912 Free PMC article.
-
Filla MS, Faralli JA, Desikan H, Peotter JL, Wannow AC, Peters DM. Filla MS, et al. Invest Ophthalmol Vis Sci. 2019 Sep 3;60(12):3897-3913. doi: 10.1167/iovs.19-27171. Invest Ophthalmol Vis Sci. 2019. PMID: 31529121 Free PMC article.
References
-
- Aguirre K.M., McCormick R.J., Schwarzbauer J.E. Fibronectin self-association is mediated by complementary sites within the amino-terminal one-third of the molecule. J. Biol. Chem. 1994;269:27863–27868. - PubMed
-
- Ali I.U., Hynes R.O. Effects of cytochalasin B and colchicine on attachment of a major surface protein of fibroblasts. Biochim. Biophys. Acta. 1977;471:16–24. - PubMed
-
- Avnur Z., Geiger B. The removal of extracellular fibronectin from areas of cell-substrate contact. Cell. 1981;25:121–132. - PubMed
-
- Bazzoni G., Hemler M.E. Are changes in integrin affinity and conformation overemphasized? Trends Biochem. Sci. 1998;23:30–34. - PubMed
MeSH terms
Substances
LinkOut - more resources
Full Text Sources
Other Literature Sources
Research Materials