Regulation of spine calcium dynamics by rapid spine motility - PubMed
- ️Sat Jan 01 2000
Regulation of spine calcium dynamics by rapid spine motility
A Majewska et al. J Neurosci. 2000.
Abstract
Dendritic spines receive most excitatory inputs in the CNS and compartmentalize calcium. Spines also undergo rapid morphological changes, although the function of this motility is still unclear. We have investigated the effect of spine movement on spine calcium dynamics with two-photon photobleaching of enhanced green fluorescent protein and calcium imaging of action potential-elicited transients in spines from layer 2/3 pyramidal neurons in mouse visual cortex slices. The elongation or retraction of the spine neck during spine motility alters the diffusional coupling between spine and dendrite and significantly changes calcium decay kinetics in spines. Our results demonstrate that the spine's ability to compartmentalize calcium is constantly changing.
Figures
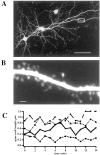
Spine neck lengths change during spine movement.A, Two-photon image of two EGFP-transfected layer 2/3 pyramidal neurons. The pial surface is to the top right.B, High-magnification image of the boxed area in A. Note how dendritic spines are clearly visible. C. Spine necks elongate and retract as they move. Analysis of the spine neck changes in four spines followed for a 15 min period. Scale bars: A, 50 μm; B, 1 μm.
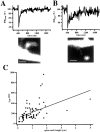
Diffusional coupling between spine and dendrite depends on the length of the spine neck. A, B, Measurements of diffusional coupling in short (A)- and long (B)-necked spines. A, Top, Fluorescence recovery curve of the spine shown in the bottom panel, whose neck length is 0.4 μm. The fitted monoexponential curve (thin line) shows a τGFP of 68 msec. B,Top, Similar measurement from a long-necked (1.5 μm) spine, with a τGFP of 275 msec. C, Relation of τGFP and spine neck length. There is a good correlation between these two variables. Scale bars, 1 μm.
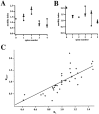
Diffusional coupling between spine and dendrite is altered as spines elongate or retract. A, B, Lack of effect of the bleaching protocol on the spine motility of bleached (A) or unbleached (B) spines on the same dendrite. Eachpanel shows the motility index of spines before (squares) and after (circles) the bleaching protocol was applied. There were no significant differences in the average motility indexes. C, Relation between theRτGFP (change in fluorescent recovery time constant) and the RL(change in spine neck length). Notice the strong correlation between the two parameters (p < 0.0001;n = 38; linear regression; correlation coefficient = 0.73) indicating that changes in the times for diffusion of EGFP through the spine neck are tied to the changes in spine neck length during motility.
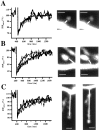
Changes in EGFP diffusion during movement in individual spines. A–C, Measurements of diffusional coupling in spines whose necks stayed the same length (A), shortened (B), or grew longer (C). Middle,Right, Images of the spine at the first time point (middle) and at the second time point (right). White lines trace the length of the neck. Left, The EGFP fluorescence recoverytraces. Solid traces correspond to the recoveries obtained at the first time point (middle), whereas stippled traces correspond to those obtained at the second time point (right). The change in time constants for the spines shown is as follows: from 130 to 135 msec (A), from 380 to 170 msec (B), and from 260 to 480 msec (C). Scale bars, 1 μm.
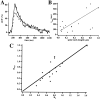
Correlation between changes in the spine neck length and changes in the fast calcium decay in spines.A, Calcium dynamics in a spine after the firing of a backpropagating action potential through an extracellular pipette placed near the cell soma. The action potential elicits an increase in calcium concentration in both dendrite and spine. The calcium decay in the dendrite (stippled line) follows single-exponential kinetics, whereas the decay in the spine (solid line) shows the characteristic double-exponential kinetics (Majewska et al., 2000a). The trace is an average of six trials, filtered with a seven-point smoothing kernel. B, Relation between spine τfast (fast time constant of decay) and spine neck length. There is a strong correlation between these two variables (p < 0.01; correlation coefficient = 0.66; n = 16). C, Relation between Rτfast andRL. Note the strong correlation between these parameters (p < 0.001;n = 16; correlation coefficient = 0.83) demonstrating that changes in spine neck length during spine movement affect the kinetics of the calcium decay in spines.
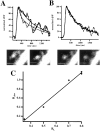
Changes in the fast calcium decay component during spine movement in individual spines. A, Example of the calcium dynamics in a spine in response to an AP. Two calcium decays from the same spine measured at different time points (top) are shown. The traces have been normalized to the same amplitude for ease of comparison. Thesolid line shows the decay that corresponds to thebottom left image of the spine. The stippled line corresponds to the bottom right image when the spine had retracted and the spine neck had become shorter. Notice the faster initial decay of the stippled trace. The second slow decay phase is similar in the two spines because of the slower and less variable dendritic decay. B, Similar analysis from a spine that did not change its neck length. Note how the calcium decay kinetics is very similar. C, Relation between Rτfast andRL for a spine in which five time points were available. The correlation is highly significant (p < 0.005; n = 5). Scale bars, 1 μm.
Similar articles
-
Majewska A, Brown E, Ross J, Yuste R. Majewska A, et al. J Neurosci. 2000 Mar 1;20(5):1722-34. doi: 10.1523/JNEUROSCI.20-05-01722.2000. J Neurosci. 2000. PMID: 10684874 Free PMC article.
-
Calcium dynamics of spines depend on their dendritic location.
Holthoff K, Tsay D, Yuste R. Holthoff K, et al. Neuron. 2002 Jan 31;33(3):425-37. doi: 10.1016/s0896-6273(02)00576-7. Neuron. 2002. PMID: 11832229
-
Kim H, Kunz PA, Mooney R, Philpot BD, Smith SL. Kim H, et al. J Neurosci. 2016 Apr 27;36(17):4888-94. doi: 10.1523/JNEUROSCI.4204-15.2016. J Neurosci. 2016. PMID: 27122043 Free PMC article.
-
Calcium dynamics in spines: link to synaptic plasticity.
Holthoff K, Tsay D. Holthoff K, et al. Exp Physiol. 2002 Nov;87(6):725-31. doi: 10.1113/eph8702463. Exp Physiol. 2002. PMID: 12530404 Review.
-
[Imaging of spatiotemporal pattern of synaptic inputs during memory replay].
Ishikawa T. Ishikawa T. Nihon Yakurigaku Zasshi. 2023;158(2):159-163. doi: 10.1254/fpj.22123. Nihon Yakurigaku Zasshi. 2023. PMID: 36858497 Review. Japanese.
Cited by
-
ROCK1 and ROCK2 inhibition alters dendritic spine morphology in hippocampal neurons.
Swanger SA, Mattheyses AL, Gentry EG, Herskowitz JH. Swanger SA, et al. Cell Logist. 2016 Jan 19;5(4):e1133266. doi: 10.1080/21592799.2015.1133266. eCollection 2015 Oct-Dec. Cell Logist. 2016. PMID: 27054047 Free PMC article.
-
Maio VD, Ventriglia F, Santillo S. Maio VD, et al. Neuronal Signal. 2017 Jun 14;1(3):NS20160051. doi: 10.1042/NS20160051. eCollection 2017 Aug. Neuronal Signal. 2017. PMID: 32714580 Free PMC article. Review.
-
González-Burgos I, Rivera-Cervantes MC, Velázquez-Zamora DA, Feria-Velasco A, Garcia-Segura LM. González-Burgos I, et al. Neural Plast. 2012;2012:309494. doi: 10.1155/2012/309494. Epub 2011 Nov 2. Neural Plast. 2012. PMID: 22164341 Free PMC article.
-
Bonthron C, Burley S, Broadhead MJ, Metodieva V, Grant SGN, Chandran S, Miles GB. Bonthron C, et al. PLoS One. 2024 Aug 1;19(8):e0306423. doi: 10.1371/journal.pone.0306423. eCollection 2024. PLoS One. 2024. PMID: 39088455 Free PMC article.
-
Anatomical and electrophysiological comparison of CA1 pyramidal neurons of the rat and mouse.
Routh BN, Johnston D, Harris K, Chitwood RA. Routh BN, et al. J Neurophysiol. 2009 Oct;102(4):2288-302. doi: 10.1152/jn.00082.2009. Epub 2009 Aug 12. J Neurophysiol. 2009. PMID: 19675296 Free PMC article.
References
-
- Brandon J, Coss R. Rapid dendritic spine stem shortening during one-trial learning: the honeybee's first orientation flight. Brain Res. 1982;252:51–61. - PubMed
-
- Crick F. Do spines twitch? Trends Neurosci. 1982;5:44–46.
Publication types
MeSH terms
Substances
LinkOut - more resources
Full Text Sources
Other Literature Sources