ATP-dependent chromatin remodeling facilitates nucleotide excision repair of UV-induced DNA lesions in synthetic dinucleosomes - PubMed
- ️Mon Jan 01 2001
ATP-dependent chromatin remodeling facilitates nucleotide excision repair of UV-induced DNA lesions in synthetic dinucleosomes
K Ura et al. EMBO J. 2001.
Abstract
To investigate the relationship between chromatin dynamics and nucleotide excision repair (NER), we have examined the effect of chromatin structure on the formation of two major classes of UV-induced DNA lesions in reconstituted dinucleosomes. Furthermore, we have developed a model chromatin-NER system consisting of purified human NER factors and dinucleosome substrates that contain pyrimidine (6-4) pyrimidone photoproducts (6-4PPs) either at the center of the nucleosome or in the linker DNA. We have found that the two classes of UV-induced DNA lesions are formed efficiently at every location on dinucleosomes in a manner similar to that of naked DNA, even in the presence of histone H1. On the other hand, excision of 6-4PPs is strongly inhibited by dinucleosome assembly, even within the linker DNA region. These results provide direct evidence that the human NER machinery requires a space greater than the size of the linker DNA to excise UV lesions efficiently. Interestingly, NER dual incision in dinucleosomes is facilitated by recombinant ACF, an ATP-dependent chromatin remodeling factor. Our results indicate that there is a functional connection between chromatin remodeling and the initiation step of NER.
Figures
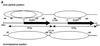
Fig. 1. The effect of chromatin structure on CPD and 6-4PP formation in 5S dinucleosome DNA. (A) Structure of the 5S dinucleosome DNA (Ura et al., 1995). The 5S dinucleosome DNA (418 bp) contains two 197 bp tandem repeats of the Xenopus borealis somatic 5S RNA gene and associated upstream sequences, including the entire nucleosome positioning element. Thick arrows indicate the location and orientation of the 120 bp 5S RNA genes. The major nucleosome positions in the absence (core particle position) or presence (chromatosome position) of linker histone are based upon our previous micrococcal nuclease mapping results and are indicated by ovals. All positions are relative to the transcription start site of the 5S RNA gene, which is denoted by +1. (B) Flow diagram showing how the UV-induced CPD and 6-4PP sites on UV-irradiated dinucleosomes were mapped. (C) Mapping of CPD and 6-4PP sites on UV-irradiated dinucleosomes. Naked DNA (lanes 1–3 and 10–12) and dinucleosomes without histone H1 (lanes 4–6 and 13–15) or with histone H1 (lanes 7–9 and 16–18) were irradiated with increasing UV doses (0, 100 or 450 J/m2) as shown at the top of the lanes. Purified DNA was digested with T4 endonuclease V to map CPD sites (lanes 1–9), or incubated with CPD photolyase followed by digestion with UVDE to map 6-4PP sites (lanes 10–18). The amount of DNA in each lane was adjusted according to the total radioactivity before performing denaturing PAGE. The position at which CPD formation is clearly inhibited by the nucleosome folding is shown by arrowheads. The rotational positioning of the 5S nucleosome was demonstrated by the hydroxyl radical cleavage pattern of dinucleosomes in lane 19. M, Maxam–Gilbert G tracks as markers. Dots indicate the the axis of dyad symmetry of the nucleosome. Vertical arrows show the location and orientation of the 5S RNA gene. Ovals indicate the predominant regions contacted by the nucleosome cores as shown in (A) (core particle position). Asterisks show the sites where synthetic 6-4PPs are introduced into the 5S dinucleosome for use as templates in the NER assay.
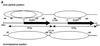
Fig. 1. The effect of chromatin structure on CPD and 6-4PP formation in 5S dinucleosome DNA. (A) Structure of the 5S dinucleosome DNA (Ura et al., 1995). The 5S dinucleosome DNA (418 bp) contains two 197 bp tandem repeats of the Xenopus borealis somatic 5S RNA gene and associated upstream sequences, including the entire nucleosome positioning element. Thick arrows indicate the location and orientation of the 120 bp 5S RNA genes. The major nucleosome positions in the absence (core particle position) or presence (chromatosome position) of linker histone are based upon our previous micrococcal nuclease mapping results and are indicated by ovals. All positions are relative to the transcription start site of the 5S RNA gene, which is denoted by +1. (B) Flow diagram showing how the UV-induced CPD and 6-4PP sites on UV-irradiated dinucleosomes were mapped. (C) Mapping of CPD and 6-4PP sites on UV-irradiated dinucleosomes. Naked DNA (lanes 1–3 and 10–12) and dinucleosomes without histone H1 (lanes 4–6 and 13–15) or with histone H1 (lanes 7–9 and 16–18) were irradiated with increasing UV doses (0, 100 or 450 J/m2) as shown at the top of the lanes. Purified DNA was digested with T4 endonuclease V to map CPD sites (lanes 1–9), or incubated with CPD photolyase followed by digestion with UVDE to map 6-4PP sites (lanes 10–18). The amount of DNA in each lane was adjusted according to the total radioactivity before performing denaturing PAGE. The position at which CPD formation is clearly inhibited by the nucleosome folding is shown by arrowheads. The rotational positioning of the 5S nucleosome was demonstrated by the hydroxyl radical cleavage pattern of dinucleosomes in lane 19. M, Maxam–Gilbert G tracks as markers. Dots indicate the the axis of dyad symmetry of the nucleosome. Vertical arrows show the location and orientation of the 5S RNA gene. Ovals indicate the predominant regions contacted by the nucleosome cores as shown in (A) (core particle position). Asterisks show the sites where synthetic 6-4PPs are introduced into the 5S dinucleosome for use as templates in the NER assay.
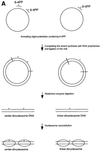
Fig. 2. Preparation and characterization of dinucleosome substrates containing synthetic 6-4PPs. (A) Schematic representation of dinucleosomes that contain 6-4PPs either in the center of the nucleosome (center-dinucleosome) or in the linker DNA (linker-dinucleosome). Asterisks indicate 6-4PPs. (B) DNase I footprinting of 6-4PP-containing dinucleosomes. Lanes 1–3, undigested DNA; lanes 4–6, digested naked DNA; lanes 7–9, digested dinucleosomes. Lane 10 shows a G-specific cleavage reaction as a marker. N, undamaged DNA (lanes 1, 4 and 7); C, center-dinucleosome DNA (lanes 2, 5 and 8); L, linker-dinucleosome DNA (lanes 3, 6 and 9). Dots indicate the axis of dyad symmetry of the nucleosome. The 10 bp repeat pattern, common to nucleosomal footprinting, is shown by arrowheads. As a control, we show that DNA fragments containing 6-4PPs are frequently slightly nicked at damage sites even without DNase I treatment (lanes 2 and 3) (Franklin et al., 1982). Dots, vertical arrows, ovals and asterisks indicate the same items described in Figure 1A. (C) Micrococcal nuclease mapping of core positions on dinucleosomes containing 6-4PPs. Dinucleosomes were digested with micrococcal nuclease. 5′-end-labeled core particle DNA (147 bp) was digested with EcoRV. There is no EcoRV site in the non-specific DNA that was used for nucleosome reconstitution with dinucleosome DNA. Lanes 1–3, undigested core particle DNA; lanes 4–6, DNA digested with EcoRV. Lane 7 shows a DNA maker produced by the hydroxyl radical cleavage reaction. N, undamaged DNA; C, center-dinucleosome DNA; L, linker-dinucleosome DNA; CP, core particle DNA products of digestion.
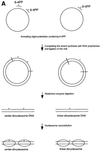
Fig. 2. Preparation and characterization of dinucleosome substrates containing synthetic 6-4PPs. (A) Schematic representation of dinucleosomes that contain 6-4PPs either in the center of the nucleosome (center-dinucleosome) or in the linker DNA (linker-dinucleosome). Asterisks indicate 6-4PPs. (B) DNase I footprinting of 6-4PP-containing dinucleosomes. Lanes 1–3, undigested DNA; lanes 4–6, digested naked DNA; lanes 7–9, digested dinucleosomes. Lane 10 shows a G-specific cleavage reaction as a marker. N, undamaged DNA (lanes 1, 4 and 7); C, center-dinucleosome DNA (lanes 2, 5 and 8); L, linker-dinucleosome DNA (lanes 3, 6 and 9). Dots indicate the axis of dyad symmetry of the nucleosome. The 10 bp repeat pattern, common to nucleosomal footprinting, is shown by arrowheads. As a control, we show that DNA fragments containing 6-4PPs are frequently slightly nicked at damage sites even without DNase I treatment (lanes 2 and 3) (Franklin et al., 1982). Dots, vertical arrows, ovals and asterisks indicate the same items described in Figure 1A. (C) Micrococcal nuclease mapping of core positions on dinucleosomes containing 6-4PPs. Dinucleosomes were digested with micrococcal nuclease. 5′-end-labeled core particle DNA (147 bp) was digested with EcoRV. There is no EcoRV site in the non-specific DNA that was used for nucleosome reconstitution with dinucleosome DNA. Lanes 1–3, undigested core particle DNA; lanes 4–6, DNA digested with EcoRV. Lane 7 shows a DNA maker produced by the hydroxyl radical cleavage reaction. N, undamaged DNA; C, center-dinucleosome DNA; L, linker-dinucleosome DNA; CP, core particle DNA products of digestion.
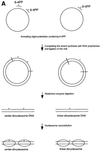
Fig. 2. Preparation and characterization of dinucleosome substrates containing synthetic 6-4PPs. (A) Schematic representation of dinucleosomes that contain 6-4PPs either in the center of the nucleosome (center-dinucleosome) or in the linker DNA (linker-dinucleosome). Asterisks indicate 6-4PPs. (B) DNase I footprinting of 6-4PP-containing dinucleosomes. Lanes 1–3, undigested DNA; lanes 4–6, digested naked DNA; lanes 7–9, digested dinucleosomes. Lane 10 shows a G-specific cleavage reaction as a marker. N, undamaged DNA (lanes 1, 4 and 7); C, center-dinucleosome DNA (lanes 2, 5 and 8); L, linker-dinucleosome DNA (lanes 3, 6 and 9). Dots indicate the axis of dyad symmetry of the nucleosome. The 10 bp repeat pattern, common to nucleosomal footprinting, is shown by arrowheads. As a control, we show that DNA fragments containing 6-4PPs are frequently slightly nicked at damage sites even without DNase I treatment (lanes 2 and 3) (Franklin et al., 1982). Dots, vertical arrows, ovals and asterisks indicate the same items described in Figure 1A. (C) Micrococcal nuclease mapping of core positions on dinucleosomes containing 6-4PPs. Dinucleosomes were digested with micrococcal nuclease. 5′-end-labeled core particle DNA (147 bp) was digested with EcoRV. There is no EcoRV site in the non-specific DNA that was used for nucleosome reconstitution with dinucleosome DNA. Lanes 1–3, undigested core particle DNA; lanes 4–6, DNA digested with EcoRV. Lane 7 shows a DNA maker produced by the hydroxyl radical cleavage reaction. N, undamaged DNA; C, center-dinucleosome DNA; L, linker-dinucleosome DNA; CP, core particle DNA products of digestion.
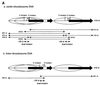
Fig. 3. Effects of chromatin structure on NER. (A) Schematic representation of the predicted cleavage products after incision of the two internally labeled 6-4PP substrates, center-dinucleosome DNA (a) and linker-dinucleosome DNA (b). The labeled products of the excision assay are represented by thin bidirectional arrows along with their lengths in nucleotides (nt). Thick arrows indicate the location and orientation of the 120 bp 5S RNA gene. The 5′-internal promoter of two repeats of the 5S RNA gene was mutated by base substitution, and is indicated by the white box in plasmid pXSP5S. Internally radiolabeled sites of center- and linker-dinucleosome DNA are indicated by black and white asterisks, respectively. The major nucleosome core positions are shown by ovals. (B) Excision activity of NER factors on synthetic chromatin templates. Chromatin templates were assembled on internally labeled DNA fragments containing either no 6-4PP (lane 1), 6-4PPs at the nucleosome centers (lanes 2–4) or a 6-4PP in the linker DNA (lanes 5–7). These were used as substrates in the excision assay. Naked DNA (lanes 1, 2 and 5), mononucleosomes (lanes 3 and 6) or dinucleosomes (lanes 4 and 7) were incubated with purified NER factors for 30 min at 30°C. Cleavage products were analyzed by 12% denaturing PAGE. Each value obtained from the excision products is expressed as a percentage of the total signal in each lane, which is shown at the bottom of the lane. This figure is representative of data obtained from repeated trials of independently conducted experiments. (C) The quantitative data from (B) shown as a bar graph. The relative excision activity of dinucleosomes containing 6-4PP at the center of nucleosomes (black bars) or in linker DNA (white bars) was calculated by taking the value of excision on each naked DNA template as 100%.
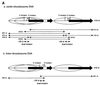
Fig. 3. Effects of chromatin structure on NER. (A) Schematic representation of the predicted cleavage products after incision of the two internally labeled 6-4PP substrates, center-dinucleosome DNA (a) and linker-dinucleosome DNA (b). The labeled products of the excision assay are represented by thin bidirectional arrows along with their lengths in nucleotides (nt). Thick arrows indicate the location and orientation of the 120 bp 5S RNA gene. The 5′-internal promoter of two repeats of the 5S RNA gene was mutated by base substitution, and is indicated by the white box in plasmid pXSP5S. Internally radiolabeled sites of center- and linker-dinucleosome DNA are indicated by black and white asterisks, respectively. The major nucleosome core positions are shown by ovals. (B) Excision activity of NER factors on synthetic chromatin templates. Chromatin templates were assembled on internally labeled DNA fragments containing either no 6-4PP (lane 1), 6-4PPs at the nucleosome centers (lanes 2–4) or a 6-4PP in the linker DNA (lanes 5–7). These were used as substrates in the excision assay. Naked DNA (lanes 1, 2 and 5), mononucleosomes (lanes 3 and 6) or dinucleosomes (lanes 4 and 7) were incubated with purified NER factors for 30 min at 30°C. Cleavage products were analyzed by 12% denaturing PAGE. Each value obtained from the excision products is expressed as a percentage of the total signal in each lane, which is shown at the bottom of the lane. This figure is representative of data obtained from repeated trials of independently conducted experiments. (C) The quantitative data from (B) shown as a bar graph. The relative excision activity of dinucleosomes containing 6-4PP at the center of nucleosomes (black bars) or in linker DNA (white bars) was calculated by taking the value of excision on each naked DNA template as 100%.
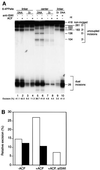
Fig. 4. Effects of rACF on NER in vitro. (A) Excision in synthetic chromatin templates. The excision reaction of NER was carried out either in the presence or absence of ACF (300 fmol) as indicated at the top of each lane. DNA fragments containing 6-4PPs in linker DNA (lanes 1–4, 9 and 10) or at the nucleosome centers (lanes 5–8) were used as substrates for the excision assay. Naked DNA (lanes 1, 2, 5, 6 and 10) or dinucleosomes (lanes 3, 4, 7, 8 and 9) were incubated with purified NER proteins in the absence (lanes 1–8) or presence (lanes 9 and 10) of antiserum against ISWI for 45 min at 30°C. Each value obtained from the excision products is expressed as a percentage of the total signal in each lane, which is shown at the bottom of the lane. This figure is representative of data obtained from repeated trials of independently conducted experiments. (B) The quantitative data from (A) shown as a bar graph. Relative excision activity of center-dinucleosomes (black bars) or linker-dinucleosomes (white bars) was calculated by taking the value of excision from each naked DNA template as 100%.
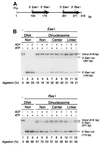
Fig. 5. Effect of rACF on accessibility of damaged DNA in dinucleosomes. (A) The locations of the EaeI and RsaI sites on 5S dinucleosome DNA. Thick arrows show the location of the 5S RNA gene. Asterisks show the 6-4PP positions. (B) Restriction enzyme digestion of dinucleosomes. 5′-end-radiolabeled naked DNA (lanes 2 and 3) and dinucleosomes (lanes 4–12) were digested with either EaeI (top) or RsaI (bottom). The restriction digestion was carried out in the presence or absence of ACF (200 fmol) and 3 mM ATP as indicated at the top of each lane. Non, undamaged DNA; Center, center-dinucleosome DNA; Linker, linker-dinucleosome DNA. The percentage digestion of the 5′ EaeI and 5′ RsaI restriction sites is shown at the bottom of each lane.
Similar articles
-
Open, repair and close again: chromatin dynamics and the response to UV-induced DNA damage.
Palomera-Sanchez Z, Zurita M. Palomera-Sanchez Z, et al. DNA Repair (Amst). 2011 Feb 7;10(2):119-25. doi: 10.1016/j.dnarep.2010.10.010. Epub 2010 Dec 3. DNA Repair (Amst). 2011. PMID: 21130713 Review.
-
UV damage and repair mechanisms in mammalian cells.
Tornaletti S, Pfeifer GP. Tornaletti S, et al. Bioessays. 1996 Mar;18(3):221-8. doi: 10.1002/bies.950180309. Bioessays. 1996. PMID: 8867736 Review.
-
Lettieri T, Kraehenbuehl R, Capiaghi C, Livingstone-Zatchej M, Thoma F. Lettieri T, et al. DNA Repair (Amst). 2008 May 3;7(5):734-43. doi: 10.1016/j.dnarep.2008.01.016. Epub 2008 Mar 10. DNA Repair (Amst). 2008. PMID: 18329964
-
Wellinger RE, Thoma F. Wellinger RE, et al. EMBO J. 1997 Aug 15;16(16):5046-56. doi: 10.1093/emboj/16.16.5046. EMBO J. 1997. PMID: 9305646 Free PMC article.
-
Thoma F. Thoma F. EMBO J. 1999 Dec 1;18(23):6585-98. doi: 10.1093/emboj/18.23.6585. EMBO J. 1999. PMID: 10581233 Free PMC article. Review.
Cited by
-
DDB2 promotes chromatin decondensation at UV-induced DNA damage.
Luijsterburg MS, Lindh M, Acs K, Vrouwe MG, Pines A, van Attikum H, Mullenders LH, Dantuma NP. Luijsterburg MS, et al. J Cell Biol. 2012 Apr 16;197(2):267-81. doi: 10.1083/jcb.201106074. Epub 2012 Apr 9. J Cell Biol. 2012. PMID: 22492724 Free PMC article.
-
The Ino80 chromatin-remodeling complex restores chromatin structure during UV DNA damage repair.
Sarkar S, Kiely R, McHugh PJ. Sarkar S, et al. J Cell Biol. 2010 Dec 13;191(6):1061-8. doi: 10.1083/jcb.201006178. Epub 2010 Dec 6. J Cell Biol. 2010. PMID: 21135142 Free PMC article.
-
Puumalainen MR, Rüthemann P, Min JH, Naegeli H. Puumalainen MR, et al. Cell Mol Life Sci. 2016 Feb;73(3):547-66. doi: 10.1007/s00018-015-2075-z. Epub 2015 Oct 31. Cell Mol Life Sci. 2016. PMID: 26521083 Free PMC article. Review.
-
Platinum anticancer drug damage enforces a particular rotational setting of DNA in nucleosomes.
Danford AJ, Wang D, Wang Q, Tullius TD, Lippard SJ. Danford AJ, et al. Proc Natl Acad Sci U S A. 2005 Aug 30;102(35):12311-6. doi: 10.1073/pnas.0506025102. Epub 2005 Aug 22. Proc Natl Acad Sci U S A. 2005. PMID: 16116097 Free PMC article.
-
Rapić-Otrin V, McLenigan MP, Bisi DC, Gonzalez M, Levine AS. Rapić-Otrin V, et al. Nucleic Acids Res. 2002 Jun 1;30(11):2588-98. doi: 10.1093/nar/30.11.2588. Nucleic Acids Res. 2002. PMID: 12034848 Free PMC article.
References
-
- Araki M. et al. (2000) Reconstitution of damage DNA excision reaction from SV40 minichromosomes with purified nucleotide excision repair proteins. Mutat. Res., 459, 147–160. - PubMed
-
- Araujo S.J., Tirode,F., Coin,F., Pospiech,H., Syvaoja,J.E., Stucki,M., Hübscher,U., Egly,J.M. and Wood,R.D. (2000) Nucleotide excision repair of DNA with recombinant human proteins: definition of the minimal set of factors, active forms of TFIIH and modulation by CAK. Genes Dev., 14, 349–359. - PMC - PubMed
-
- Batty D.P. and Wood,R.D. (2000) Damage recognition in nucleotide excision repair of DNA. Gene, 241, 193–204. - PubMed
-
- de Laat W.L., Jaspers,N.G. and Hoeijmakers,J.H. (1999) Molecular mechanism of nucleotide excision repair. Genes Dev., 13, 768–785. - PubMed
Publication types
MeSH terms
Substances
LinkOut - more resources
Full Text Sources