Cytosolic acidification as a signal mediating hyperosmotic stress responses in Dictyostelium discoideum - PubMed
Cytosolic acidification as a signal mediating hyperosmotic stress responses in Dictyostelium discoideum
T Pintsch et al. BMC Cell Biol. 2001.
Abstract
Background: Dictyostelium cells exhibit an unusual response to hyperosmolarity that is distinct from the response in other organisms investigated: instead of accumulating compatible osmolytes as it has been described for a wide range of organisms, Dictyostelium cells rearrange their cytoskeleton and thereby build up a rigid network which is believed to constitute the major osmoprotective mechanism in this organism. To gain more insight into the osmoregulation of this amoeba, we investigated physiological processes affected under hyperosmotic conditions in Dictyostelium.
Results: We determined pH changes in response to hyperosmotic stress using FACS or 31P-NMR. Hyperosmolarity was found to acidify the cytosol from pH 7.5 to 6.8 within 5 minutes, whereas the pH of the endo-lysosomal compartment remained constant. Fluid-phase endocytosis was identified as a possible target of cytosolic acidification, as the inhibition of endocytosis observed under hypertonic conditions can be fully attributed to cytosolic acidification. In addition, a deceleration of vesicle mobility and a decrease in the NTP pool was observed.
Conclusion: Together, these results indicate that hyperosmotic stress triggers pleiotropic effects, which are partially mediated by a pH signal and which all contribute to the downregulation of cellular activity. The comparison of our results with the effect of hyperosmolarity and intracellular acidification on receptor-mediated endocytosis in mammalian cells reveals striking similarities, suggesting the hypothesis of the same mechanism of inhibition by low internal pH.
Figures
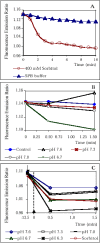
FACS measurements using BCECF as fluorescent pH indicator show that hyperosmotic stress results in intracellular acidification (A) AX-2 cells were labeled with BCECF and were subsequently exposed to hyperosmotic stress. Fluorescence emission was determined at FL1 and FL2 by flow cytometry and the fluorescence emission ratio FL1/FL2 was calculated. The decrease in FL1/FL2 of cells exposed to hyperosmotic shock ("400 mM Sorbitol") reflects a decrease in pH. As control, the measurements were performed with cells suspended in SPB buffer. (B) Calibration of intracellular pH of cells in low osmolarity buffer. BCECF-labeled cells were suspended in calibration solutions with different Pseudo-Null pH values [25] and FL1/FL2 was determined by flow cytometry at intervals. The solution with Pseudo-Null pH 7.3 slightly decreased FL1/FL2 compared to the control cells suspended in SPB buffer and the solution with Pseudo-Null pH 7.6 increases FL1/FL2 compared to the control. Hence, the actual intracellular pH is estimated to be pH 7.3 to pH 7.4. (C) Calibration of the pH of hyperosmotically shocked cells. After BCECF labeling, Dictyostelium cells were suspended SPB buffer and FL1/FL2 was determined at T=-13.5 min. Sorbitol solution was added (indicated by the upper arrow) and the cells were incubated for 10 min. The cells were centrifuged and resuspended in high osmolarity calibration solutions with different Pseudo-Null pH values at T = 0 min (indicated by the lower arrow). FL1/FL2 was determined by flow cytometry at intervals. The solution with Pseudo-Null pH 6.7 slightly decreased FL1/FL2 compared to the control ("Sorbitol"; cells suspended in SPB buffer/400 mM sorbitol) at T = 0.5 min, before intracellular pH starts to recover. This suggests, that the actual intracellular pH is 6.7-6.8. The points of time specified in Fig. 1C are not drawn to scale.
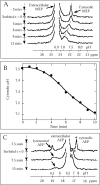
In vivo31 P-NMR analysis of the effect of hyperosmotic shock on cytosolic pH in Dictyostelium cells (A) The sequence of 31P-NMR spectra represents a typical experiment conducted with the probe MEP. After addition of MEP and preincubation during 60 min, a control spectrum was recorded for 3 min immediately before sorbitol addition (upper trace). Sorbitol (0.3 M) was then added at T = 0 and data were collected during successive 3-min intervals up to 12 min. NMR spectra shown are the sum of 180 free induction decays with 1-s interpulse delays and correspond to a 3-min accumulation. Only the spectral region around +24 ppm corresponding to MEP probe is shown. The pH scale shown on abcissa was calculated from the in vivo calibration curve pH = 7.6 + log(24.5 - δ)/(δ - 20.6), 8 being the chemical shift of MEP resonance. (B) Temporal evolution of cytosolic pH after onset of hyperosmotic shock in Dictyostelium cells calculated from the position of cytosolic MEP resonance (see A). (C) In vivo31P-NMR analysis of the effect of hyperosmotic shock on lysosomal pH in Dictyostelium cells. The sequence of successive 31P-NMR spectra represents a typical experiment conducted with the probe AEP. After addition of AEP and preincubation for 60 min, a control spectrum was recorded for 7.5 min immediately before sorbitol addition (upper trace). Sorbitol (0.3 M) was then added at T = 0 min and data were collected during two consecutive 7.5-min intervals. Only the spectral region around +18 ppm corresponding to AEP probe is shown. The pH scale indicated on the graph was calculated from the in vivo AEP calibration curve pH = 6.3 + log(19.1 - δ)/(δ - 16.5), δ being the chemical shift of AEP resonance. Lysosomal and cytosolic AEP satellite peaks, lowfield and upfield of extracellular AEP, respectively, are indicated by arrow and star symbols, respectively.
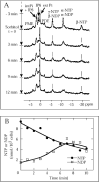
In vivo31 P-NMR analysis of the effect of osmotic shock on phosphorylated metabolites in Dictyostelium cells (A) The experiments were performed as described for the determination of cytosolic pH by 31P-NMR using MEP as probe. Only the spectral region (+5 to -20 ppm) corresponding to phosphorylated metabolites is shown. (B) Temporal evolution of intracellular nucleoside di- and triphosphate content during hyperosmotic shock in Dictyostelium cells. The relative intracellular NTP content was calculated from the area of the P-NTP peak at about -20 ppm and the NDP content was deduced from the area of the (β-NDP + γNTP) peak at about -5 ppm. The NTP and NDP content (nmol/107 cells) before hyperosmotic shock (values at T = 0) were measured on perchloric acid extracts. Abb.: PME, phosphomonoesters; PDE, phosphodiesters; cytPi, extPi, cytosolic and extracellular Pi; NTP, NDP, nucleoside tri- and diphosphate; IP6, inositol hexakisphosphate.
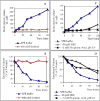
Hyperosmotic stress results in the abolishment of endo- and exocytosis partially due to cytosolic acidification (A) Cells which were resuspended in SPB buffer avidly endocytose the fluorescent fluid-phase marker FITC-Dextran, whereas no fluid uptake was observed with cells exposed to 400 mM sorbitol in SPB buffer. (B) Correspondingly, cells in SPB buffer rapidly exocytosed ingested FITC-Dextran, whereas no loss of fluorescence was measured with cell suspended in 400 mM sorbitol, indicating that exocytosis is blocked. (C) Cells, which were artificially acidified by suspending the cells in SPB buffer/5 mM propionic acid (pH 6.0)/FITC-Dextran did not accumulate any FITC-Dextran, indicating that endocytosis was blocked. Also suspending the cells in SPB buffer/30 μM DES/FITC-Dextran resulted in the inhibition of endocytosis. However, a resumption of endocytic activity was observed after 1 h of hyperosmotic shock, presumably due to adaptation. As control, cells were suspended in SPB buffer/FITC-Dextran. (D) Artificial acidification of cells partially inhibits exocytosis. Control cells labeled with FITC-Dextran exocytosed about 90% of the dye within 2 h, whereas a loss of 55%-60% of cell-associated fluorescence was measured with cells exposed to 5 mM propionic acid or 30 μM DES.
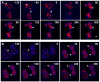
Vesicle mobility and membrane flow are downregulated under hypertonic conditions. Cells were either labeled with TRITC-Dextran (A) or with Cy3.5 (B). Points of time at which confocal images were scanned are indicated in seconds referring to the onset of hyperosmotic shock as T = 0. (A) The fluorescent label of TRITC-Dextran in red is superimposed to phase contrast images in blue. TRITC-Dextran labeled vesicles of cells suspended in SPB buffer are mobile (indicated by arrows in frames -120, -60). Upon sorbitol shock, perturbation and immobilization of vesicles was observed. As an example, a region with stalled and distorted vesicles is indicated by arrows in frames 60-300. (B) The fluorescent label of Cy3.5 in red is superimposed to phase contrast images in blue. Cells suspended in SPB buffer occassionally endocytose membrane (arrows in frames -120, -60). Upon hyperosmotic shock, the plasma membrane thickens and forms spikes after cell shrinkage. The spikes are not endocytosed but remain fixed. Typical spikes are indicated with arrows in frames 30-300. Bar = 2 μm.
Similar articles
-
Brénot F, Aubry L, Martin JB, Satre M, Klein G. Brénot F, et al. Biochimie. 1992 Sep-Oct;74(9-10):883-95. doi: 10.1016/0300-9084(92)90072-m. Biochimie. 1992. PMID: 1281673
-
Hisactophilin is involved in osmoprotection in Dictyostelium.
Pintsch T, Zischka H, Schuster SC. Pintsch T, et al. BMC Biochem. 2002 May 7;3:10. doi: 10.1186/1471-2091-3-10. BMC Biochem. 2002. PMID: 11996675 Free PMC article.
-
The endocytic pathways of Dictyostelium discoideum.
Vines JH, King JS. Vines JH, et al. Int J Dev Biol. 2019;63(8-9-10):461-471. doi: 10.1387/ijdb.190236jk. Int J Dev Biol. 2019. PMID: 31840784 Review.
-
Conserved features of endocytosis in Dictyostelium.
Maniak M. Maniak M. Int Rev Cytol. 2002;221:257-87. doi: 10.1016/s0074-7696(02)21014-1. Int Rev Cytol. 2002. PMID: 12455750 Review.
Cited by
-
Endocytic response of type I alveolar epithelial cells to hypertonic stress.
Wang S, Singh RD, Godin L, Pagano RE, Hubmayr RD. Wang S, et al. Am J Physiol Lung Cell Mol Physiol. 2011 Apr;300(4):L560-8. doi: 10.1152/ajplung.00309.2010. Epub 2011 Jan 21. Am J Physiol Lung Cell Mol Physiol. 2011. PMID: 21257731 Free PMC article.
-
From Pinocytosis to Methuosis-Fluid Consumption as a Risk Factor for Cell Death.
Ritter M, Bresgen N, Kerschbaum HH. Ritter M, et al. Front Cell Dev Biol. 2021 Jun 23;9:651982. doi: 10.3389/fcell.2021.651982. eCollection 2021. Front Cell Dev Biol. 2021. PMID: 34249909 Free PMC article. Review.
-
Visualizing myosin-actin interaction with a genetically-encoded fluorescent strain sensor.
Iwai S, Uyeda TQ. Iwai S, et al. Proc Natl Acad Sci U S A. 2008 Nov 4;105(44):16882-7. doi: 10.1073/pnas.0805513105. Epub 2008 Oct 29. Proc Natl Acad Sci U S A. 2008. PMID: 18971336 Free PMC article.
-
Agglomeration: when folded proteins clump together.
Romero-Romero ML, Garcia-Seisdedos H. Romero-Romero ML, et al. Biophys Rev. 2023 Dec 15;15(6):1987-2003. doi: 10.1007/s12551-023-01172-4. eCollection 2023 Dec. Biophys Rev. 2023. PMID: 38192350 Free PMC article. Review.
-
The arrestin-domain containing protein AdcA is a response element to stress.
Habourdin C, Klein G, Araki T, Williams JG, Aubry L. Habourdin C, et al. Cell Commun Signal. 2013 Nov 22;11:91. doi: 10.1186/1478-811X-11-91. Cell Commun Signal. 2013. PMID: 24267687 Free PMC article.
References
-
- Yancey HP, Dark ME, Hand SC, Bowlus RD, Somero GN. Living with Water Stress: Evolution of Osmolyte Systems. Science. 1982;217:1214–1222. - PubMed
-
- Baumgarten CM, Feher JJ. Osmosis and the regulation of cell volume. In Cell Physiology Source Book Edited by Sperelakis N pp 180-211 San Diego, CA: Academic Press; 1995.
-
- Ruis H, Schuller C. Stress signaling in yeast. BioEssays. 1995;17:959–965. - PubMed
-
- Cohen D, Wassermann J, Gullans S. Immediate early gene and HSP70 expression in hyperosmotic stress in MDCK cells. American Journal of Physiology. 1991;261:C594–C601. - PubMed
Publication types
MeSH terms
Substances
LinkOut - more resources
Full Text Sources