The human heart and rat brain IIA Na+ channels interact with different molecular regions of the beta1 subunit - PubMed
The human heart and rat brain IIA Na+ channels interact with different molecular regions of the beta1 subunit
Thomas Zimmer et al. J Gen Physiol. 2002 Dec.
Abstract
The alpha subunit of voltage-gated Na(+) channels of brain, skeletal muscle, and cardiomyocytes is functionally modulated by the accessory beta(1), but not the beta(2) subunit. In the present study, we used beta(1)/beta(2) chimeras to identify molecular regions within the beta(1) subunit that are responsible for both the increase of the current density and the acceleration of recovery from inactivation of the human heart Na(+) channel (hH1). The channels were expressed in Xenopus oocytes. As a control, we coexpressed the beta(1)/beta(2) chimeras with rat brain IIA channels. In agreement with previous studies, the beta(1) extracellular domain sufficed to modulate IIA channel function. In contrast to this, the extracellular domain of the beta(1) subunit alone was ineffective to modulate hH1. Instead, the putative membrane anchor plus either the intracellular or the extracellular domain of the beta(1) subunit was required. An exchange of the beta(1) membrane anchor by the corresponding beta(2) subunit region almost completely abolished the effects of the beta(1) subunit on hH1, suggesting that the beta(1) membrane anchor plays a crucial role for the modulation of the cardiac Na(+) channel isoform. It is concluded that the beta(1) subunit modulates the cardiac and the neuronal channel isoforms by different molecular interactions: hH1 channels via the membrane anchor plus additional intracellular or extracellular regions, and IIA channels via the extracellular region only.
Figures
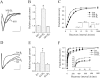
Modulation of hH1 and IIA Na+ channels by the β1 subunit. (A) Representative current traces for hH1 and hH1/β1 channels at the test potentials −10 and −30 mV. The τh values of hH1 versus hH1/β1 channels were statistically indistinguishable (−30 mV: τh = 2.21 ± 0.18 for hH1, τh = 2.01 ± 0.43 for hH1/β1 [P = 0.64]; −10 mV: τh = 1.25 ± 0.07 for hH1, τh = 1.12 ± 0.08 for hH1/β1 [P = 0.23]). Number of experiments: n = 11 for hH1, n = 6 for hH1/β1. Calibration bars = 4 ms, at −30 mV: 0.25 μA for hH1, 0.62 μA for hH1/β1; at −10 mV: 0.45 μA for hH1, 1.06 μA for hH1/β1. (B) Effect of the β1 subunit on the hH1 peak current amplitude in Xenopus oocytes (*P < 0.001). Currents were measured 3 d after injection at the test potential of −25 mV. Measurements were performed in seven different batches of oocytes. Data from a single batch of oocytes were normalized with respect to the mean current of hH1-injected oocytes (n = 63 for hH1, n = 50 for hH1/β1, and n = 52 for hH1/β2). (C) Time course of recovery from inactivation of hH1 channels. The respective voltage protocol is shown in the inset (n = 32 for hH1, n = 38 for hH1/β1, and n = 21 for hH1/β2). (D) Effect of the β1 subunit on the inactivation time course of rat brain IIA Na+ currents. The Na+ currents were elicited by a test pulse to −10 mV, and normalized with respect to the peak current. Calibration bars = 5 ms, 0.9 μA for IIA, 0.8 μA for IIA/β1, and 0.7 μA for IIA/β2. Statistically, the inactivation time constant τh was not different for IIA and IIA/β2 channels at the applied test pulses (unpublished data). (E) Effect of the β1 subunit on the IIA peak current amplitude (*P < 0.001). Currents were measured 3 d after injection at the test potential of −10 mV (n = 13 for IIA, n = 13 for IIA/β1 and n = 13 for IIA/β2). (F) Time course of recovery from inactivation of IIA channels. Currents were elicited by the same voltage protocol as indicated in C, except that a test pulse to −10 mV was used (n = 7 for IIA, n = 7 for IIA/β1 and n = 6 for IIA/β2). Bars indicate SEM.
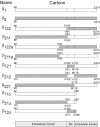
Structure of the chimeras between the Na+ channel β1 and β2 subunits used in this study. The corresponding terminal amino acids of the β1 (white boxes) and β2 (gray boxes) subunit regions are indicated. The assumed topology of both subunits in the plasma membrane is shown below the cartoons of the chimeras.
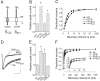
Modulatory effect of chimeras β122 and β211 on hH1 and IIA channels. (A) Schematic representation of the domain structures of both chimeras. (B) Relative current amplitudes (test pulse to −25 mV; *P < 0.001; n = 55 for hH1, n = 37 for hH1/β1, n = 38 for hH1/β122, and n = 50 for hH1/β211). (C) Time course of recovery from inactivation of hH1 channels (n = 7 for hH1, n = 6 for hH1/β1, n = 5 for hH1/β122, and n = 6 for hH1/β211). (D) Effect of β122 on the inactivation time course of rat brain IIA Na+ currents (test pulse: −10 mV). Calibration bars = 5 ms, 0.25 μA for IIA, 0.52 μA for IIA/β1, 0.27 μA for IIA/β122, and 0.21 μA for IIA/β211. (E) Effect of β122 on the IIA peak current amplitude (test pulse to −10 mV; *P < 0.001; n = 19 for IIA, n = 13 for IIA/β1, n = 19 for IIA/β122, and n = 17 for IIA/β211). (F) Time course of recovery from inactivation of IIA channels. For voltage protocol, see legend to Fig. 1 (n = 13 for IIA, n = 12 for IIA/β1 and n = 12 for IIA/β122, and n = 12 for IIA/β211). Bars indicate SEM.
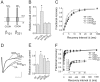
Coexpression of chimeras β121 and β221 with hH1 and IIA channels. (A) Schematic representation of the domain structures of both chimeras. (B) Relative current amplitudes (test pulse to −25 mV; *P < 0.001; n = 29 for hH1, n = 17 for hH1/β1, n = 28 for hH1/β121, and n = 21 for hH1/β221). (C) Time course of recovery from inactivation of hH1 channels (n = 21 for hH1, n = 17 for hH1/β1, n = 12 for hH1/β121, and n = 19 for hH1/β221). (D) Effect of β121 on the time course of inactivation of rat brain IIA Na+ currents (test pulse to −10 mV). Calibration bars = 5 ms, 0.93 μA for IIA, 0.90 μA for IIA/β1, 0.62 μA for IIA/β121, and 0.90 μA for IIA/β221. (E) Effect of β121 and β221 on the IIA peak current amplitude (test pulse to −10 mV; *P < 0.001; n = 15 for IIA, n = 9 for IIA/β1, n = 12 for IIA/β121, and n = 19 for IIA/β221). (F) Time course of recovery from inactivation of IIA channels (n = 13 for IIA, n = 9 for IIA/β1 and n = 5 for IIA/β121, and n = 13 for IIA/β221). Bars indicate SEM.
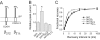
Coexpression of chimera β212 and β21Δ with hH1 channels. (A) Schematic representation of the domain structure of β212 and β21Δ. (B) Relative current amplitudes (test pulse to −25 mV; *P < 0.001; n = 17 for hH1, n = 10 for hH1/β1, n = 21 for hH1/β212, and n = 11 for β21Δ). (C) Time course of recovery from inactivation of hH1 channels (n = 10 for hH1, n = 4 for hH1/β1, n = 11 for hH1/β212, and n = 7 for β21Δ). Bars indicate SEM.
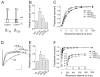
Coexpression of β11Δ and β12Δ with hH1 and IIA channels. (A) Schematic representation of the domain structures of both deletion variants. (B) Relative current amplitudes (test pulse to −25 mV; *P < 0.001; n = 38 for hH1, n = 38 for hH1/β1, n = 30 for hH1/β11Δ, and n = 23 for hH1/β12Δ). (C) Time course of recovery from inactivation of hH1 channels (n = 23 for hH1, n = 21 for hH1/β1, n = 19 for hH1/β11Δ, and n = 20 for hH1/β12Δ). (D) Effect of β11Δ and β12Δ on the inactivation time course of rat brain IIA Na+ currents (test pulse to −10 mV). Calibration bars = 5 ms, 0.92 μA for IIA, 0.76 μA for IIA/β1, 0.80 μA for IIA/β11Δ, and 0.28 μA for IIA/β12Δ. (E) Effect of β11Δ and β12Δ on the IIA peak current amplitude (test pulse to −10 mV; *P < 0.001; n = 16 for IIA, n = 14 for IIA/β1, n = 21 for IIA/β11Δ, and n = 9 for IIA/β12Δ). (F) Time course of recovery from inactivation of IIA channels (n = 13 for IIA, n = 13 for IIA/β1, n = 16 for IIA/β11Δ, and n = 10 for IIA/β12Δ). Bars indicate SEM.
Similar articles
-
Nuss HB, Chiamvimonvat N, Pérez-García MT, Tomaselli GF, Marbán E. Nuss HB, et al. J Gen Physiol. 1995 Dec;106(6):1171-91. doi: 10.1085/jgp.106.6.1171. J Gen Physiol. 1995. PMID: 8786355 Free PMC article.
-
Makita N, Bennett PB, George AL Jr. Makita N, et al. J Neurosci. 1996 Nov 15;16(22):7117-27. doi: 10.1523/JNEUROSCI.16-22-07117.1996. J Neurosci. 1996. PMID: 8929421 Free PMC article.
-
McCormick KA, Srinivasan J, White K, Scheuer T, Catterall WA. McCormick KA, et al. J Biol Chem. 1999 Nov 12;274(46):32638-46. doi: 10.1074/jbc.274.46.32638. J Biol Chem. 1999. PMID: 10551818
-
Structural determinants of slow inactivation in human cardiac and skeletal muscle sodium channels.
Vilin YY, Makita N, George AL Jr, Ruben PC. Vilin YY, et al. Biophys J. 1999 Sep;77(3):1384-93. doi: 10.1016/S0006-3495(99)76987-0. Biophys J. 1999. PMID: 10465750 Free PMC article.
-
Molecular properties of brain sodium channels: an important target for anticonvulsant drugs.
Catterall WA. Catterall WA. Adv Neurol. 1999;79:441-56. Adv Neurol. 1999. PMID: 10514834 Review.
Cited by
-
Mutations in sodium channel β1- and β2-subunits associated with atrial fibrillation.
Watanabe H, Darbar D, Kaiser DW, Jiramongkolchai K, Chopra S, Donahue BS, Kannankeril PJ, Roden DM. Watanabe H, et al. Circ Arrhythm Electrophysiol. 2009 Jun;2(3):268-75. doi: 10.1161/CIRCEP.108.779181. Epub 2009 Mar 6. Circ Arrhythm Electrophysiol. 2009. PMID: 19808477 Free PMC article.
-
Wu M, Ye N, Sengupta B, Zakon HH. Wu M, et al. J Comp Physiol A Neuroethol Sens Neural Behav Physiol. 2013 Oct;199(10):829-42. doi: 10.1007/s00359-013-0845-3. Epub 2013 Aug 25. J Comp Physiol A Neuroethol Sens Neural Behav Physiol. 2013. PMID: 23979192
-
Binary architecture of the Nav1.2-β2 signaling complex.
Das S, Gilchrist J, Bosmans F, Van Petegem F. Das S, et al. Elife. 2016 Feb 19;5:e10960. doi: 10.7554/eLife.10960. Elife. 2016. PMID: 26894959 Free PMC article.
-
Identification of Navβ1 residues involved in the modulation of the sodium channel Nav1.4.
Islas AA, Sánchez-Solano A, Scior T, Millan-PerezPeña L, Salinas-Stefanon EM. Islas AA, et al. PLoS One. 2013 Dec 16;8(12):e81995. doi: 10.1371/journal.pone.0081995. eCollection 2013. PLoS One. 2013. PMID: 24358138 Free PMC article.
-
Baroni D, Barbieri R, Picco C, Moran O. Baroni D, et al. J Bioenerg Biomembr. 2013 Aug;45(4):353-68. doi: 10.1007/s10863-013-9510-3. Epub 2013 Apr 13. J Bioenerg Biomembr. 2013. PMID: 23584539
References
-
- Auld, V.J., A.L. Goldin, D.S. Krafte, J. Marshall, J.M. Dunn, W.A. Catterall, H.A. Lester, N. Davidson, and R.J. Dunn. 1988. A rat brain Na+ channel α subunit with novel gating properties. Neuron. 1:449–461. - PubMed
-
- Catterall, W.A. 1992. Cellular and molecular biology of voltage-gated sodium channels. Physiol. Rev. 72:S15–S48. - PubMed
-
- Chauhan, V.S., S. Tuvia, M. Buhusi, V. Bennett, and A.O. Grant. 2000. Abnormal cardiac Na+ channel properties and QT heart rate adaptation in neonatal ankyrinB knockout mice. Circ. Res. 86:441–447. - PubMed
-
- Chen, C., and S.C. Cannon. 1995. Modulation of Na+ channel inactivation by the β1 subunit: a deletion analysis. Pflugers Arch. 431:186–195. - PubMed
Publication types
MeSH terms
Substances
LinkOut - more resources
Full Text Sources
Research Materials