Identification of vascular lineage-specific genes by transcriptional profiling of isolated blood vascular and lymphatic endothelial cells - PubMed
Identification of vascular lineage-specific genes by transcriptional profiling of isolated blood vascular and lymphatic endothelial cells
Satoshi Hirakawa et al. Am J Pathol. 2003 Feb.
Abstract
In mammals, the lymphatic vascular system develops by budding of lymphatic progenitor endothelial cells from embryonic veins to form a distinct network of draining vessels with important functions in the immune response and in cancer metastasis. However, the lineage-specific molecular characteristics of blood vascular versus lymphatic endothelium have remained poorly defined. We isolated lymphatic endothelial cells (LECs) and blood vascular endothelial cells (BVECs) by immunomagnetic isolation directly from human skin. Cultured LECs but not BVECs expressed the lymphatic markers Prox1 and LYVE-1 and formed LYVE-1-positive vascular tubes after implantation in vivo. Transcriptional profiling studies revealed increased expression of several extracellular matrix and adhesion molecules in BVECs, including versican, collagens, laminin, and N-cadherin, and of the growth factor receptors endoglin and vascular endothelial growth factor receptor-1/Flt-1. Differential immunostains of human skin confirmed the blood vessel-specific expression of these genes. During embryonic development, endoglin expression was gradually down-regulated on lymphatic endothelium whereas vascular endothelial growth factor receptor-1 was absent from lymphatics. We also identified several genes with specific expression in LECs. These results demonstrate that some lineage-specific genes are only expressed during distinct developmental stages and they identify new molecular markers for blood vascular and lymphatic endothelium with important implications for future studies of vascular development and function.
Figures
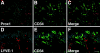
Specific detection of lymphatic and blood vessels in normal human skin. A–C: The homeobox gene Prox1 is specifically expressed by LECs (A, red), whereas blood vessels express CD34 (B, green). Double immunostains for Prox1 (red) and CD34 (green) reveal mutually exclusive vascular expression in lymphatic and blood vessels (C). Nuclei are labeled blue (Hoechst stain). D–F: Specific staining of lymphatic vessels with an antibody against the hyaluronan receptor LYVE-1 (D, red) and of blood vessels with an anti-CD34 antibody (E, green). F: Double immunostains for LYVE-1 (red) and CD34 (green) demonstrate mutually exclusive expression in lymphatics and blood vessels. Scale bar, 100 μm.
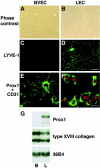
Cultured human dermal LECs and BVECs maintain their lineage-specific differentiation in vitro. A and B: Cultured BVECs and LECs (passage 4) show comparable endothelial morphology with characteristic cobblestone appearance. Phase-contrast micrograph. C and D: All LECs express the lymphatic marker LYVE-1 in vitro (D), whereas BVECs are not labeled (C). E and F: Double immunostains for CD31 (green) and Prox1 (red) demonstrate CD31 membrane labeling in both cell types whereas Prox1 (nuclear stain, red) is selectively expressed in LECs. G: Northern blot analysis confirms selective Prox1 mRNA expression in cultured LECs (L), whereas no expression is found in BVECs (B). In contrast, the basement membrane component type XVIII collagen is expressed at higher levels in BVECs. Hybridization with a probe to ribosomal protein-associated RNA 36B4 demonstrates equal loading. Scale bars: 100 μm (D); 25 μm (F).
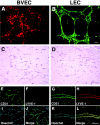
BVECs and LECs maintain lineage-specific differentiation after formation of tube-like structures in vivo. A and B: Cultured BVECs and LECs were fluorescently labeled with CMTMR (orange) or CMFDA (green), respectively, and were seeded onto Matrigel. Both BVECs (A) and LECs (B) demonstrate formation of tube-like structures in vitro, a characteristic feature of endothelial cells. C–L: Isolated BVECs or LECs were mixed with Matrigel and were implanted subcutaneously into SCID mice. Efficient formation of tube-like structures by BVECs (C) and LECs (D) after 7 days in vivo. H&E stains. Both BVECs (E) and LECs (G) express CD31 (green) in vivo, whereas only tubes formed by LECs (H), but not by BVECs (F) are also positive for LYVE-1 (red). I and K: Nuclear stain with Hoechst (blue). J and L: Double immunostains for CD31 (green) and LYVE-1 (red), combined with nuclear stains (blue), confirm lineage-specific tube formation in Matrigel. Scale bars: 50 μm (B); 100 μm (D, L).
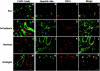
Blood vessel-specific expression of VEGFR-1/Flt1, N-cadherin, versican, and endoglin in normal human skin. Differential immunostains reveal mutually exclusive expression of LYVE-1 (red) on lymphatic vessels and of VEGFR-1/Flt-1 (A), N-cadherin (E), versican (I), and endoglin (M) on cutaneous blood vessels (green). Nuclear Hoechst counterstain (blue) was used to depict skin morphology. Double immunostains for the pan-endothelial marker CD31 (red) and selected blood vascular markers (green) demonstrate that only a fraction of all vessels also express VEGFR-1/Flt1 (B–D), N-cadherin (F–H), versican (J–L), or endoglin (N–P). Scale bar, 50 μm.
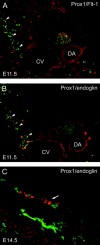
Expression of endoglin and VEGFR-1/Flt-1 during embryonic development of the lymphatic vascular system. Throughout the embryonic development of the lymphatic vascular system, Flt-1 is expressed selectively on BVECs, whereas endoglin is expressed on both blood vascular and LECs. A: Flt-1 expression (red) is limited to the vascular endothelium at E11.5 and is particularly high on endothelial cells of the dorsal aorta (DA). In contrast, Flt-1 is not detected on LECs located in or budding from (arrowheads) the cardinal vein (CV). Lymphatic endothelial cells are identified by the expression of Prox1 (green). B: In an adjacent section, endoglin expression (red) is detected on both blood vascular and LECs. C: At E14.5, endoglin is strongly expressed on blood vessels (green), whereas lymphatic vessels show reduced expression (arrow, detected by expression of Prox1, red).
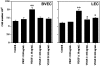
LEC and BVEC proliferation was equally stimulated by VEGF-A. Both VEGF-C and VEGF-D induced LEC proliferation with only minor effects on BVECs. Mean values ± SD. *, P < 0.05; **, P < 0.01.
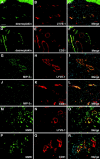
Lymphatic endothelium-specific expression of desmoplakin, CCL20/MIP-3α and macrophage mannose receptor in normal human skin. A–C: All desmoplakin-positive lymphatic vessels also express LYVE-1, whereas only a fraction of CD31-positive vessels also expresses desmoplakin (D–F). G–I: Co-localization of MIP-3α and LYVE-1 in cutaneous lymphatic vessels. J–L: Blood vessels (red in L) do not express MIP-3α. M–O: Expression of macrophage mannose receptor (MMR) is detected on all LYVE-1-positive small lymphatic vessels (orange in O), whereas MMR expression is absent from blood vessels (P–R); blood vessels are depicted in red in R. Scale bar, 50 μm.
Similar articles
-
Kriehuber E, Breiteneder-Geleff S, Groeger M, Soleiman A, Schoppmann SF, Stingl G, Kerjaschki D, Maurer D. Kriehuber E, et al. J Exp Med. 2001 Sep 17;194(6):797-808. doi: 10.1084/jem.194.6.797. J Exp Med. 2001. PMID: 11560995 Free PMC article.
-
Prox1 is a master control gene in the program specifying lymphatic endothelial cell fate.
Hong YK, Harvey N, Noh YH, Schacht V, Hirakawa S, Detmar M, Oliver G. Hong YK, et al. Dev Dyn. 2002 Nov;225(3):351-7. doi: 10.1002/dvdy.10163. Dev Dyn. 2002. PMID: 12412020
-
Mäkinen T, Veikkola T, Mustjoki S, Karpanen T, Catimel B, Nice EC, Wise L, Mercer A, Kowalski H, Kerjaschki D, Stacker SA, Achen MG, Alitalo K. Mäkinen T, et al. EMBO J. 2001 Sep 3;20(17):4762-73. doi: 10.1093/emboj/20.17.4762. EMBO J. 2001. PMID: 11532940 Free PMC article.
-
Prox1, master regulator of the lymphatic vasculature phenotype.
Hong YK, Detmar M. Hong YK, et al. Cell Tissue Res. 2003 Oct;314(1):85-92. doi: 10.1007/s00441-003-0747-8. Epub 2003 Jul 22. Cell Tissue Res. 2003. PMID: 12883994 Review.
-
Key molecules in lymphatic development, function, and identification.
Jha SK, Rauniyar K, Jeltsch M. Jha SK, et al. Ann Anat. 2018 Sep;219:25-34. doi: 10.1016/j.aanat.2018.05.003. Epub 2018 May 26. Ann Anat. 2018. PMID: 29842991 Review.
Cited by
-
Aguilar B, Choi I, Choi D, Chung HK, Lee S, Yoo J, Lee YS, Maeng YS, Lee HN, Park E, Kim KE, Kim NY, Baik JM, Jung JU, Koh CJ, Hong YK. Aguilar B, et al. Cancer Res. 2012 Nov 15;72(22):5833-42. doi: 10.1158/0008-5472.CAN-12-1229. Epub 2012 Aug 31. Cancer Res. 2012. PMID: 22942256 Free PMC article.
-
Radiogenic lymphangiogenesis in the skin.
Jackowski S, Janusch M, Fiedler E, Marsch WC, Ulbrich EJ, Gaisbauer G, Dunst J, Kerjaschki D, Helmbold P. Jackowski S, et al. Am J Pathol. 2007 Jul;171(1):338-48. doi: 10.2353/ajpath.2007.060589. Am J Pathol. 2007. PMID: 17591978 Free PMC article.
-
In Vitro, In Vivo, and In Silico Models of Lymphangiogenesis in Solid Malignancies.
Bekisz S, Baudin L, Buntinx F, Noël A, Geris L. Bekisz S, et al. Cancers (Basel). 2022 Mar 16;14(6):1525. doi: 10.3390/cancers14061525. Cancers (Basel). 2022. PMID: 35326676 Free PMC article. Review.
-
From tumor lymphangiogenesis to lymphvascular niche.
Hirakawa S. Hirakawa S. Cancer Sci. 2009 Jun;100(6):983-9. doi: 10.1111/j.1349-7006.2009.01142.x. Epub 2009 Feb 20. Cancer Sci. 2009. PMID: 19385973 Free PMC article. Review.
-
Walking the Line: A Fibronectin Fiber-Guided Assay to Probe Early Steps of (Lymph)angiogenesis.
Mitsi M, Schulz MM, Gousopoulos E, Ochsenbein AM, Detmar M, Vogel V. Mitsi M, et al. PLoS One. 2015 Dec 21;10(12):e0145210. doi: 10.1371/journal.pone.0145210. eCollection 2015. PLoS One. 2015. PMID: 26689200 Free PMC article.
References
-
- Witte MH, Bernas MJ, Martin CP, Witte CL: Lymphangiogenesis and lymphangiodysplasia: from molecular to clinical lymphology. Microsc Res Tech 2001, 55:122-145 - PubMed
-
- Oliver G, Detmar M: The rediscovery of the lymphatic system. Old and new insights into the development and biological function of the lymphatic vascular system. Genes Dev 2002, 16:773-783 - PubMed
-
- Saeki H, Moore AM, Brown MJ, Hwang ST: Cutting edge: secondary lymphoid-tissue chemokine (SLC) and CC chemokine receptor 7 (CCR7) participate in the emigration pathway of mature dendritic cells from the skin to regional lymph nodes. J Immunol 1999, 162:2472-2475 - PubMed
-
- Alitalo K, Carmeliet P: Molecular mechanisms of lymphangiogenesis in health and disease. Cancer Cell 2002, 1:219-227 - PubMed
-
- Skobe M, Hawighorst T, Jackson DG, Prevo R, Janes L, Velasco P, Riccardi L, Alitalo K, Claffey K, Detmar M: Induction of tumor lymphangiogenesis by VEGF-C promotes breast cancer metastasis. Nat Med 2001, 7:192-198 - PubMed
Publication types
MeSH terms
Substances
Grants and funding
LinkOut - more resources
Full Text Sources
Other Literature Sources
Research Materials
Miscellaneous