Microtubules provide directional cues for polarized axonal transport through interaction with kinesin motor head - PubMed
- ️Wed Jan 01 2003
Microtubules provide directional cues for polarized axonal transport through interaction with kinesin motor head
Takao Nakata et al. J Cell Biol. 2003.
Abstract
Post-Golgi carriers of various newly synthesized axonal membrane proteins, which possess kinesin (KIF5)-driven highly processive motility, were transported from the TGN directly to axons. We found that KIF5 has a preference to the microtubules in the initial segment of axon. Low dose paclitaxel treatment caused missorting of KIF5, as well as axonal membrane proteins to the tips of dendrites. Microtubules in the initial segment of axons showed a remarkably high affinity to EB1-YFP, which was known to bind the tips of growing microtubules. These findings revealed unique features of the microtubule cytoskeletons in the initial segment, and suggested that they provide directional information for polarized axonal transport.
Figures
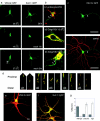
Post-Golgi transport of axonal and dendrite carriers in hippocampal neurons visualized by CLSM. (a) Sorting of membrane protein–GFP fusion proteins under specific cell manipulation. VSVtsO45-G::GFP localizes in the ER at 39.5°C and moves to the TGN after a 30-min incubation at 19.5°C. Its post-Golgi transport starts when the temperature is shifted to 37°C. Note that VSV-G::GFP carriers are predominantly transported to one neurite (arrows) among the others. Kv2.1::GFP distributes diffusely in the somatodendritic area when expressed overnight in the presence of 1 μM brefeldin A. Kv2.1::GFP accumulates at the Golgi region 1 h after the brefeldin A washout, and starts post-Golgi transport. Note that Kv2.1::GFP was transported to all the dendrites 3 h after wash. (b) Characterization of VSV-G::G(Y)FP probes in neurons. (b1) Neurons were infected with Ad(VSVtsO45-G::GFP) and incubated overnight at 39.5°C, for 30 min at 19.5°C, and for 1 h at 37°C in the presence of 1 mg/ml Texas-red dextran (MW3000). Note that post-Golgi VSV-G carriers in the axon (arrows, green) are not labeled with the endocytotic marker (red). (b2 and b3) Double-label of hippocampal neuron with VSV-G::YFP and Golgi–CFP. At 19.5°C (b2), VSV-G::YFP (b2, green) was colocalized with the Golgi complex marker (b2, red) in the cell body. When the temperature is shifted to 37°C (b3), VSV-G::YFP moves from the Golgi complex area (yellow area at the upper right of b3, due to the overlap of VSV-G::YFP [green] and Golgi–CFP [red]) to the axon (b3, arrows). (c) VSV-G::GFP was dominantly transported from the TGN to the axon (arrow). Neurons were infected with Ad(VSVtsO45-G::GFP) and incubated overnight at 39.5°C, for 30 min at 19.5°C, and for 1 h at 37°C to visualize post-Golgi membrane transport (green). After fixation, the dendrites were stained with the anti-MAP2 antibody (red). Bar, 50 μm. Videos 1 and 2 are available at
http://www.jcb.org/cgi/content/full/jcb.200302175/DC1. (d) Post-Golgi axonal carriers of VSV-G::GFP transport various membrane proteins. Axonally transported tubulovesicular organelles were simultaneously double-labeled with CFP- and YFP-tagged proteins, and time-lapse data were collected by sequential activation with 442 and 488 nm lasers by CLSM. (d1) VSV-G::CFP::CFP (red) and GAP-43::YFP (green). (d2) VSV-G::CFP::CFP (red) and β-APP::YFP (green). (d3) VSV-G::YFP (green) and Vamp2::CFP (red). (d4) Vamp2::CFP (red) and GAP-43::YFP (green). (d5) Vamp2::CFP (red) and β-APP::YFP (green). In each set, interval between the right and left panel is 10 s. Slight gaps between the CFP and YFP images along the longitudinal axis of vesicles are due to the time lag (∼0.7 s) between the sequential data acquisition. (e and f) Dominant negative kinesin (S205A,H206A; stained with the H2 antibody; red) inhibits polarized axonal transport of VSV-G::GFP (e, green), whereas it does not inhibit significantly dendrite transport of Kv2.1::GFP (f, green). Bars, 10 μm. (g) Inhibition of polarized axonal transport by dominant negative kinesins. Black bars indicate percentage of neurons with polarized VSV-G::GFP transport, and white bars indicate percentage of neurons exhibiting accumulation of VSV-G::GFP at TGN. Data were collected from four independent cultures.
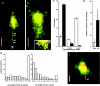
Analysis of axonal and dendrite carrier movement by CAFM. CAFM images of the post-Golgi complex carriers to the axon (a: VSV-G::GFP) and to the dendrites (b: Kv2.1::GFP). Two images at 10-s intervals were merged into one image to show the displacements of individual carriers for 10 s (red images are obtained 10 s later than the green images). Axonal tubulovesicular organelles showed high motility and dominantly transported to the axon (arrows in a indicate three moving carriers). In contrast, post-Golgi carriers for Kv2.1 were vesicular in shape and do not show directional preference in motility (inset in b shows a high magnification of the area indicated by an arrow. Three moving carriers are indicated by arrows in the inset. Note carriers with displacements less than their diameter show overlapping yellow region of two merged images). Corresponding videos are available as Videos 3–5. (c–e) Quantitative analysis of the dynamics of axonal versus dendrite carriers. (c) Percentage of the carriers which shows 0 μm, <1 μm, >1 μm displacements in 10 s in the cell body. Black bar, Kv2.1 carriers; white bar, VSV-G carriers. Data were obtained from three independent cultures. 200 carriers were counted in each culture. (d) Number of the post-Golgi carriers remaining within the cell body. Carriers were counted at 1 h after the start of the post-Golgi transport. Black bar, Kv2.1 carriers; white bar, VSV-G carriers. (e) Histogram of the run-length of individual 100 post-Golgi carriers of Kv2.1 and VSV-G. Carriers, which showed no displacement, were omitted from the histogram. Carriers, which move into neurites and get out of the observing field, were included in the group of >5 μm run-length. (f) Simultaneous double labeling with Kv2.1::YFP (green) and VSV-G::CFP::CFP (red). Note that they colocalize in the Golgi complex area. Kv2.1 carriers distributed in the somatodendritic area, but VSV-G carriers move to the IS (arrow). Bars, 10 μm.
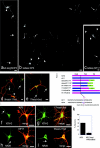
Tailless motors were destined for different regions of neurons. Data were obtained by CLSM. (a) Full-length KIF5B::GFP was transfected into hippocampal neurons. It was distributed throughout the cell body, dendrites, and the axon (arrows). The cell body and dendrites were brightly stained, just because of their thickness compared with the axon. (b) Tailless KIF5B::YFP were transfected into hippocampal neurons. Tailless KIF5B::YFP accumulated at the tips of axonal branches (arrows) but not dendrite tips. Cell body and proximal axon are indicated by arrowheads. (c) Tailless KIF17::YFP accumulated at the tips of dendrites (arrowheads) and axons (arrow). (d and e) Experiments on exchange of head domains revealed that head domains determine the destination of the tailless motors. MAP2 antibody is used as dendrite marker. (d) The KIF5B head and the KIF17 neck and rod constructs were destined for axons (green, arrows). The arrowhead indicates the cell body of transfected neuron. (e) The KIF17 head and the KIF5B neck and rod constructs were destined for the tips of dendrites (green). (f and g) Head exchange affects on polarized axonal transport. (f) Polarized axonal transport of VSV-G::GFP (green) was analyzed in the neurons that overexpress full-length KIF5B (red). VSV-G::GFP was preferentially sorted to the axon (arrow). (g) Preferential sorting of VSV-G::GFP (green, arrows) to the axon was inhibited in the neurons, which overexpress full-length KIF5 whose motor domain was replaced with KIF17 (red; see corresponding Videos 6 and 7). Fixation and immunocytochemistry to detect motor proteins was performed after live-cell imaging of VSV-G::GFP transport. (h and i) Head exchange does not affect on polarized dendrite sorting. NR2B::YFP distribution (green) was examined in the neurons, which overexpress authentic KIF17 (h, red) and full-length KIF17 whose motor domain was replaced with KIF5 (i, red). NR2B (green) was not sorted into axons in both cases. Arrows indicated the most likely candidates for axons. Bars, 10 μm. (j) Schematic diagram of the constructs. Head was exchanged at the border of head and neck-linker region of KIF5, and the corresponding region of KIF17 by the alignment. The colored amino acid sequences in the exchanged area were highly conserved. (k) Inhibition of polarized axonal transport by the head-exchanged KIF5. Bars indicate percentage of neurons with polarized β-APP::YFP transport. Data were collected from three independent cultures.
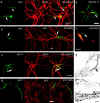
KIF5 is preferentially recruited to the MTs in the IS. After brief expression of rigor mutants of motor proteins, cells were permeabilized, fixed, and stained with antibodies. CLSM images of the strongly bound motors were obtained by serial Z-sectioning with a small confocal aperture to avoid the effect of the thickness of the cells. Data are presented as a maximal Z-projection image. Arrows indicate the IS. (a and b) Neurons expressing tailless KIF5B G234A::YFP (green) were stained with anti-MAP2 antibody (a, red) or with anti–β-tubulin antibody (b, red). Center of the cell body and the IS were intensely labeled by rigor-KIF5. (c and d) Neurons expressing tailless KIF17 G243A::YFP (green) were stained with anti-MAP2 antibody (c, red) and with anti–β-tubulin antibody (d, red). Dendrites as well as the IS (c, arrow) were intensely labeled with rigor-KIF17. (e) Simultaneous double-labeling with rigor-KIF5 (red) and tailless rigor-KIF17::YFP (green). KIF5 was stained with antikinesin antibody H2. Although the IS was intensely labeled by the both motors (arrow), KIF5 preferentially labels the center of the cell body, whereas KIF17 labels dendrites as well as axons. (f) Simultaneous double-labeling with rigor-KIF5 (green) and rigor-KIF2 (red). Although the labeling pattern in the cell body was similar in both KIFs, KIF5 preferentially labels the IS (arrow), whereas rigor-KIF2 does not. Bars, 10 μm. (g–i) Immuno-electron microscopy of rigor-KIF5 in hippocampal neurons. Rigor-KIF5 was labeled with 5-nm colloidal gold followed by silver enhancement. (g) Intense labeling of the IS is observable even at this low magnification (arrow). C indicates the cell body. (h) Higher magnification of the dendrite area in g (box D). (i) Higher magnification of axon area in g (box A). Note that extensive labeling of axons compared with dendrites. Bar, 500 nm.
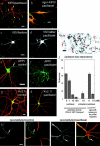
Paclitaxel at a low dose reverts the polarized sorting of axonal motor proteins as well as membrane proteins. Data were obtained by CLSM with small pinhole and maximal Z-projection (b, k, l, m, and n) and with pinhole fully open (a, c, d, e, f, g, and h). Arrows indicate axons. MAP2 (red) was stained in a, b, e, l, and m. (a) Tailless KIF5::YFP (green) was sorted to dendrite tips in the presence of 10 nM paclitaxel. (b) Rigor-KIF5::YFP (green) failed to accumulate in the IS in the presence of 10 nM paclitaxel. (c and d) VSV-G::GFP was initially observed 1 h after the temperature shift to 30°C from 19.5°C without paclitaxel (c), and 10 nM paclitaxel (d) was added to the medium. Axonal transport from the IS was unchanged. However, as a result of the decrease in the supply of vesicles from TGN, the amount of vesicles in the IS markedly decreased 1 h later (d, arrow). Note that dendrite staining is elevated in d. (e and f) β-APP::YFP was expressed overnight without (e) or with paclitaxel (f). (g and h) Kv2.1::YFP was expressed overnight without (g) or with paclitaxel (h). Kv2.1 (green) is a potassium channel sorted to the cell body and dendrites in the absence (g) and in the presence of paclitaxel (h). MAP2 was stained with red. Bars, 10 μm. (i) MT polarity is mixed in proximal dendrites after incubation with 100 nM paclitaxel overnight. (inset) Axonal MTs. Polarity of MTs is determined by the curvature of hooks in the electron micrographs (arrows). Bar, 100 nm. Electron micrograph, which shows MT organization in paclitaxel treated neurons, is available as Fig. S3. (j) Dose dependence of membrane phenotype and that of motor phenotype are similar. For membrane phenotype, percentage of neurons, which show polarized axonal transport of VSV-G::GFP are presented (n = 3, each 50 cells counted). For motor phenotype, percentage of neurons, which show preferential binding of rigor-KIF5 to the IS are presented (n = 2, each 50 cells counted). (k–n) In vitro reconstitution of preferential association of KIF5 to the MTs in the IS. Hippocampal neuronal cytoskeleton was prepared by permeabilization with 0.1% Triton X-100, and recombinant tailless-KIF5::YFP was added to the cytoskeleton (k and m). Subsequently, the cells were fixed and stained with anti-MAP2 antibody. In l and n, MAP2 staining (red) is superimposed on the KIF5 images in k and m (green). In control neurons, intense binding of KIF5 to the IS was observed (k and l, arrows), compared with other neurites. In contrast, when neurons were pretreated with 10 nM paclitaxel before permeabilization, binding of KIF5 to the axonal IS was similar to other neurites (m and n, arrows).
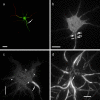
MT dynamics in the IS. (a) Maximal Z-projection of small pinhole size CLSM images of neuron transfected with EB1::YFP (green) was stained with anti-MAP2 antibody (red). EB1 extensively labeled the IS (arrow). (b and c) CAFM images of EB1::YFP expressing neurons. (b) Individual EB1 dots, which decorate the growing tips of MTs are clearly observed in dendrites, cell body, and axon in a low level of EB1::YFP expression. Note that individual EB1 dots are resolved in the IS (b, arrows; Video 8). (c) In a higher level of expression, EB1::YFP extensively labeled the MTs in the IS (c, arrow), whereas it labeled only tips of MTs in the cell body and dendrites (c, arrowheads; Video 9). (d) CAFM image of MTs stained with antitubulin antibody. Note that MTs in dendrites as well as cell bodies are visualized by CAFM. Arrow indicates MTs in the cell body. Bars, 10 μm.
Similar articles
-
Karasmanis EP, Phan CT, Angelis D, Kesisova IA, Hoogenraad CC, McKenney RJ, Spiliotis ET. Karasmanis EP, et al. Dev Cell. 2018 Jul 16;46(2):204-218.e7. doi: 10.1016/j.devcel.2018.06.013. Dev Cell. 2018. PMID: 30016622 Free PMC article.
-
Analyzing kinesin motor domain translocation in cultured hippocampal neurons.
Yang R, Bentley M, Huang CF, Banker G. Yang R, et al. Methods Cell Biol. 2016;131:217-232. doi: 10.1016/bs.mcb.2015.06.021. Epub 2015 Sep 3. Methods Cell Biol. 2016. PMID: 26794516 Free PMC article.
-
Tubulin tyrosination navigates the kinesin-1 motor domain to axons.
Konishi Y, Setou M. Konishi Y, et al. Nat Neurosci. 2009 May;12(5):559-67. doi: 10.1038/nn.2314. Epub 2009 Apr 19. Nat Neurosci. 2009. PMID: 19377471
-
Differentiation between Oppositely Oriented Microtubules Controls Polarized Neuronal Transport.
Tas RP, Chazeau A, Cloin BMC, Lambers MLA, Hoogenraad CC, Kapitein LC. Tas RP, et al. Neuron. 2017 Dec 20;96(6):1264-1271.e5. doi: 10.1016/j.neuron.2017.11.018. Epub 2017 Nov 30. Neuron. 2017. PMID: 29198755 Free PMC article. Review.
-
Smart motors and cargo steering drive kinesin-mediated selective transport.
Nabb AT, Frank M, Bentley M. Nabb AT, et al. Mol Cell Neurosci. 2020 Mar;103:103464. doi: 10.1016/j.mcn.2019.103464. Epub 2020 Jan 20. Mol Cell Neurosci. 2020. PMID: 31972342 Free PMC article. Review.
Cited by
-
Mechanisms of Polarized Organelle Distribution in Neurons.
Britt DJ, Farías GG, Guardia CM, Bonifacino JS. Britt DJ, et al. Front Cell Neurosci. 2016 Mar 31;10:88. doi: 10.3389/fncel.2016.00088. eCollection 2016. Front Cell Neurosci. 2016. PMID: 27065809 Free PMC article. Review.
-
Posttranslational modifications of tubulin and the polarized transport of kinesin-1 in neurons.
Hammond JW, Huang CF, Kaech S, Jacobson C, Banker G, Verhey KJ. Hammond JW, et al. Mol Biol Cell. 2010 Feb 15;21(4):572-83. doi: 10.1091/mbc.e09-01-0044. Epub 2009 Dec 23. Mol Biol Cell. 2010. PMID: 20032309 Free PMC article.
-
Yenjerla M, LaPointe NE, Lopus M, Cox C, Jordan MA, Feinstein SC, Wilson L. Yenjerla M, et al. J Alzheimers Dis. 2010;19(4):1377-86. doi: 10.3233/JAD-2010-1335. J Alzheimers Dis. 2010. PMID: 20061604 Free PMC article.
-
Synaptic vesicle proteins are selectively delivered to axons in mammalian neurons.
Watson ET, Pauers MM, Seibert MJ, Vevea JD, Chapman ER. Watson ET, et al. Elife. 2023 Feb 2;12:e82568. doi: 10.7554/eLife.82568. Elife. 2023. PMID: 36729040 Free PMC article.
-
Guardia CM, De Pace R, Sen A, Saric A, Jarnik M, Kolin DA, Kunwar A, Bonifacino JS. Guardia CM, et al. PLoS Biol. 2019 May 17;17(5):e3000279. doi: 10.1371/journal.pbio.3000279. eCollection 2019 May. PLoS Biol. 2019. PMID: 31100061 Free PMC article.
References
-
- Ahmari, S.E., J. Buchanan, and S.J. Smith. 2000. Assembly of presynaptic active zones from cytoplasmic transport packets. Nat. Neurosci. 3:445–451. - PubMed
-
- Banker, G.A., and W.M. Cowan. 1977. Rat hippocampal neurons in dispersed cell culture. Brain Res. 126:397–442. - PubMed
-
- Burack, M.A., M.A. Silverman, and G. Banker. 2000. The role of selective transport in neuronal protein sorting. Neuron. 26:465–472. - PubMed
-
- Coy, D.L., W.O. Hancock, M. Wagenbach, and J. Howard. 1999. Kinesin's tail domain is an inhibitory regulator of the motor domain. Nat. Cell Biol. 1:288–292. - PubMed
Publication types
MeSH terms
Substances
LinkOut - more resources
Full Text Sources
Other Literature Sources
Miscellaneous