Complex receptive fields in primary visual cortex - PubMed
Review
Complex receptive fields in primary visual cortex
Luis M Martinez et al. Neuroscientist. 2003 Oct.
Abstract
In the early 1960s, Hubel and Wiesel reported the first physiological description of cells in cat primary visual cortex. They distinguished two main cell types: simple cells and complex cells. Based on their distinct response properties, they suggested that the two cell types could represent two consecutive stages in receptive-field construction. Since the 1960s, new experimental and computational evidence provided serious alternatives to this hierarchical model. Parallel models put forward the idea that both simple and complex receptive fields could be built in parallel by direct geniculate inputs. Recurrent models suggested that simple cells and complex cells may not be different cell types after all. To this day, a consensus among hierarchical, parallel, and recurrent models has been difficult to attain; however, the circuitry used by all models is becoming increasingly similar. The authors review theoretical and experimental evidence for each line of models emphasizing their strengths and weaknesses.
Figures
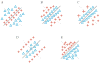
Simple receptive fields (colored version of figure 2 from Hubel and Wiesel, 1962). Red crosses represent on-subregions and blue triangles off-subregions.
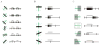
Three different types of complex receptive fields (modified version of figures 4, 6 and 7 of Hubel and Wiesel, 1962). Complex cells are a very heterogeneous population.. Cell A generates on-off responses throughout the entire receptive field. Cell B responds exclusively to a black horizontal bar. Cell C has partially separated on- and off-regions but the receptive field cannot be mapped with small spots of light. The green icons on the left represent the complex receptive field with the stimuli (flashed bars or borders) overlaid.
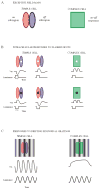
Simple and complex cells differ in their receptive-field structure and their responses to static and moving stimuli. A, Idealized receptive-field maps of a simple cell and a complex cell. B, Cartoon of the intracellular responses from a simple cell to small spots (light/dark) presented on each subregion (left and middle, the traces show a push-pull arrangement of excitatory and inhibitory inputs in the simple receptive field). Complex cells lack segregated on and off regions, small spots (light/dark) evoke on and off (push-push) responses throughout the entire receptive field (right). C, Cartoon representing the responses of a simple cell and a complex cell to drifting sinusoidal gratings.
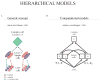
Hierarchical models put forth the idea that simple cells and complex cells represent two successive stages of cortical processing. A, Simple receptive fields are generated in layer 4 from the convergent input of geniculate neurons with receptive fields aligned in visual space. Complex receptive fields are, in turn, generated in the superficial layers by pooling the input from layer 4 simple cells with similar orientation preferences. B, Newer versions of the model incorporate more elaborated intracortical circuitry. E and I stand for excitatory and inhibitory neurons respectively. θ, orientation; k, spatial frequency, φ, spatial phase. Full details are given in the text.
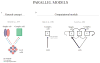
A, Parallel models advocate that simple cells and complex cells are both created in parallel from different thalamocortical pathways. B, The most recent versions of parallel models come in two sorts that exploit the preponderance of the single cells over the circuit (Mel et al’s) or vice versa (Tao et al’s). Mel et al’s proposal generates complex-cell responses that are orientation selective through specific intradendritic computations. Tao et al’s scheme obtains simple cells and complex cells by modulating the gain of the thalamocortical and corticocortical inputs. The line thickness represents the strength of the connection. E and I stand for excitatory and inhibitory neurons respectively. See the text for details.
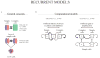
Recurrent models changed the focus of attention from single cells to networks of cortical connections. A, Most models are inspired by Douglas and Martin’s canonical microcircuit that emphasized the predominance of the input from intracortical, connections over the thalamocortical input (bottom). The most recent addenda to the family of recurrent models are the so-called state-dependent models. State-dependent models propose that simple and complex cells originate from the same cortical circuit operating at different amplification gains (top). B, Debanne et al’s model (left), emphasizes the balance between excitatory and inhibitory inputs as the origin of different cortical receptive fields. Chance et al’s model (right), emphasizes the strength of local excitatory connections within the superficial layers of the cortex. The line thickness represents the strength of the connection. E and I stand for excitatory and inhibitory neurons respectively. A more detailed explanation is given in the text.
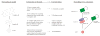
Hubel-and-Wiesel’s hierarchical model (left) has been extensively challenged over the years. Two main criticisms (middle) have propelled the emergence of alternative frameworks that accounted for the new experimental findings. Here, we propose a new circuit diagram for cat primary visual cortex (right) that embraces all current ideas of hierarchical, parallel and recurrent models. Layer 4 simple-cells and superficial complex-cells form two successive stages in receptive field construction. In addition, some cells in layer 4 have complex receptive fields derived, in parallel, from direct geniculate inputs. At each layer, local intracortical circuits (both excitatory and inhibitory) modulate the gain of the network. See the text for a detailed explanation.
Similar articles
-
Mapping receptive fields in primary visual cortex.
Ringach DL. Ringach DL. J Physiol. 2004 Aug 1;558(Pt 3):717-28. doi: 10.1113/jphysiol.2004.065771. Epub 2004 May 21. J Physiol. 2004. PMID: 15155794 Free PMC article. Review.
-
Construction of complex receptive fields in cat primary visual cortex.
Martinez LM, Alonso JM. Martinez LM, et al. Neuron. 2001 Nov 8;32(3):515-25. doi: 10.1016/s0896-6273(01)00489-5. Neuron. 2001. PMID: 11709161
-
Hierarchical models of object recognition in cortex.
Riesenhuber M, Poggio T. Riesenhuber M, et al. Nat Neurosci. 1999 Nov;2(11):1019-25. doi: 10.1038/14819. Nat Neurosci. 1999. PMID: 10526343
-
Receptive fields and response properties of neurons in layer 4 of ferret visual cortex.
Usrey WM, Sceniak MP, Chapman B. Usrey WM, et al. J Neurophysiol. 2003 Feb;89(2):1003-15. doi: 10.1152/jn.00749.2002. J Neurophysiol. 2003. PMID: 12574476 Free PMC article.
-
Thalamocortical specificity and the synthesis of sensory cortical receptive fields.
Alonso JM, Swadlow HA. Alonso JM, et al. J Neurophysiol. 2005 Jul;94(1):26-32. doi: 10.1152/jn.01281.2004. J Neurophysiol. 2005. PMID: 15985693 Review.
Cited by
-
Construction of direction selectivity through local energy computations in primary visual cortex.
Lochmann T, Blanche TJ, Butts DA. Lochmann T, et al. PLoS One. 2013;8(3):e58666. doi: 10.1371/journal.pone.0058666. Epub 2013 Mar 15. PLoS One. 2013. PMID: 23554913 Free PMC article.
-
A computational theory of visual receptive fields.
Lindeberg T. Lindeberg T. Biol Cybern. 2013 Dec;107(6):589-635. doi: 10.1007/s00422-013-0569-z. Epub 2013 Nov 7. Biol Cybern. 2013. PMID: 24197240 Free PMC article.
-
Keator DB, Fallon JH, Lakatos A, Fowlkes CC, Potkin SG, Ihler A; Alzheimer's Disease Neuroimaging Initiative. Keator DB, et al. Hum Brain Mapp. 2014 Jan;35(1):38-52. doi: 10.1002/hbm.22149. Epub 2012 Jul 30. Hum Brain Mapp. 2014. PMID: 22847891 Free PMC article.
-
Ursino M, La Cara GE. Ursino M, et al. J Comput Neurosci. 2005 Dec;19(3):291-310. doi: 10.1007/s10827-005-2491-4. J Comput Neurosci. 2005. PMID: 16284912
-
Su C, Mendes-Platt RF, Alonso JM, Swadlow HA, Bereshpolova Y. Su C, et al. J Neurosci. 2024 May 8;44(19):e1945232024. doi: 10.1523/JNEUROSCI.1945-23.2024. J Neurosci. 2024. PMID: 38485258 Free PMC article.
References
-
- Abbott LF, Chance FS. Rethinking the taxonomy of visual neurons. Nat Neurosci. 2002;5:391–392. - PubMed
-
- Adelson EH, Bergen JR. Spatiotemporal energy models for the perception of motion. J Opt Soc Am A. 1985;2:284–299. - PubMed
-
- Ahmed B, Anderson JC, Douglas RJ, Martin KA, Nelson JC. Polyneuronal innervation of spiny stellate neurons in cat visual cortex. Journal of Comparative Neurology. 1994;341:39–49. - PubMed
-
- Allison JD, Kabara JF, Snider RK, Casagrande VA, Bonds AB. GABA B-receptor-mediated inhibition reduces the orientation selectivity of the sustained response of striate cortical neurons in cats. Vis Neurosci. 1996;13:559–566. - PubMed
Publication types
MeSH terms
LinkOut - more resources
Full Text Sources
Miscellaneous