Differential targeting of two distinct SWI/SNF-related Drosophila chromatin-remodeling complexes - PubMed
Differential targeting of two distinct SWI/SNF-related Drosophila chromatin-remodeling complexes
Lisette Mohrmann et al. Mol Cell Biol. 2004 Apr.
Abstract
The SWI/SNF family of ATP-dependent chromatin-remodeling factors plays a central role in eukaryotic transcriptional regulation. In yeast and human cells, two subclasses have been recognized: one comprises yeast SWI/SNF and human BAF, and the other includes yeast RSC and human PBAF. Therefore, it was puzzling that Drosophila appeared to contain only a single SWI/SNF-type remodeler, the Brahma (BRM) complex. Here, we report the identification of two novel BRM complex-associated proteins: Drosophila Polybromo and BAP170, a conserved protein not described previously. Biochemical analysis established that Drosophila contains two distinct BRM complexes: (i) the BAP complex, defined by the presence of OSA and the absence of Polybromo and BAP170, and (ii) the PBAP complex, containing Polybromo and BAP170 but lacking OSA. Determination of the genome-wide distributions of OSA and Polybromo on larval salivary gland polytene chromosomes revealed that BAP and PBAP display overlapping but distinct distribution patterns. Both complexes associate predominantly with regions of open, hyperacetylated chromatin but are largely excluded from Polycomb-bound repressive chromatin. We conclude that, like yeast and human cells, Drosophila cells express two distinct subclasses of the SWI/SNF family. Our results support a close reciprocity of chromatin regulation by ATP-dependent remodelers and histone-modifying enzymes.
Figures
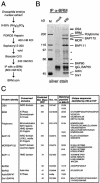
Purification and characterization of the BRM complex from Drosophila embryo nuclear extracts. (A) Outline of the chromatographic scheme used to purify Drosophila BRM and associated proteins. IP, immunoprecipitation; α-BRM, antibodies to BRM; BRM.com, Brahma complex. (B) Polypeptide composition of the BRM complex. Peak fractions from the Bioscale Q10 column were combined and incubated with beads coated with affinity-purified anti-BRM antibodies. Mock-treated beads were used as a negative control. Proteins retained on the beads after extensive washes with a buffer containing 800 mM KCl and 0.1% NP-40 were resolved by SDS-PAGE on 8% polyacrylamide gels and stained with silver stain. The nine abundant proteins that consistently copurified with BRM are designated. Degradation products are indicated by asterisks. No polypeptides smaller than 45 kDa were detected on gels with a higher percentage of polyacrylamide (data not shown). Lane M, marker proteins; lane #36, fraction 36. IgG, immunoglobulin G. (C) Summary of BRM-associated proteins identified by MS. Experimental details are described in Materials and Methods. The conserved domains and the predicted molecular (Mol.) masses of the identified proteins are indicated. The numbers of tryptic peptides out of the total identified by MALDI-TOF peptide mass fingerprinting which gave unique matches are shown, along with the GenBank accession numbers (Acc. no.) of the corresponding gene loci; multip, multiprotein. Unique sequence tags identified through tandem MS sequencing by ESI with a Q-TOF instrument (ESI-Q-TOF) also are shown.
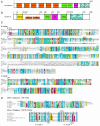
Domain structures of Drosophila Polybromo and BAP170. (A) Schematic representation of the functional domains of D. melanogaster (dm) Polybromo (GenBank accession no. NP_651288). Abbreviations: Bromo, bromodomain; Zn C2H2, C2H2 zinc finger motif. (B) Schematic representation of the domain structure of D. melanogaster (dm) BAP170. Indicated are the ARID region, consensus and variant LXXLL sequence motifs (where L is leucine and X is any amino acid) implicated in binding to hormone receptors or coactivators, domains rich in proline and glutamine residues (P/Q), a serine-rich region (S), and two putative C2H2 zinc finger motifs. (C) Alignment of the conserved sequence domains of Drosophila BAP170 (accession no. NM_136372) with homologous predicted amino acid sequences of A. gambiae (accession no. EAA04740), C. elegans (accession no. NP_495679), Homo sapiens (homo sap; accession no. XM_292131 and XP_300559), and Mus musculus (mm; accession no. BAB24929, XM_128029.3, and BAC28898) identified by a BLAST search with the full-length BAP170 sequence. The major conserved blocks in the N- and C-terminal (term) parts of the proteins are shown. Indicated are the conserved ARID region (boxed sequence), several consensus and variant LXXLL sequence motifs, and the potential zinc-coordinating residues (marked with asterisks) of canonical and variant C2H2 zinc finger motifs. Amino acids are given in the single-letter code, and conserved residues are color coded (hydrophobic, blue; acidic, orange; basic, red; polar, green; and glycine or proline, yellow). Please note that a complete human sequence is not available. (D) Sequence alignment of the ARID region and zinc finger domain of BAP170 with cognate structures from other Drosophila (dm), human (hs), S. cerevisiae (sc), Ictalurus punctatus (ip), and mouse (mm) proteins. Residues involved in coordinating zinc are marked with asterisks. Sequence alignments and structural domain predictions were performed with
http://smart.embl-heidelberg.de/smart/show_motifs.pland
http://www.ebi.ac.uk/clustalw/.
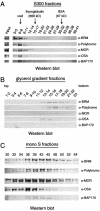
Polybromo and BAP170 are present in a high-molecular-mass complex. (A) Drososphila embryo nuclear extracts were fractionated by Sephacryl S-300 size-exclusion chromatography. The indicated fractions were combined and resolved by SDS-PAGE, followed by immunoblotting with antibodies (α) directed against BRM, Polybromo, MOR, OSA, and BAP170. All of these BRM-associated proteins were present in fractions corresponding to molecular masses of 2 MDa or greater. The elution of the voided volume (void) and the elution of the known markers thyroglobulin (669 kDa) and bovine serum albumin (BSA) (67 kDa) are indicated. (B) Embryo nuclear extracts were centrifuged through a glycerol gradient, and the different fractions collected were examined for the presence of BRM, Polybromo, MOR, OSA, and BAP170 by immunoblotting. (C) The pooled Bioscale Q10 fractions containing BRM were purified further by Mono-S column chromatography. A relevant selection of the fractions analyzed by immunoblotting with antibodies directed against BRM, Polybromo, MOR, OSA, and BAP170 is shown.
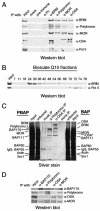
Polybromo and OSA define two distinct BRM chromatin-remodeling complexes. (A) Drosophila embryo nuclear extracts were incubated with control protein A beads (mock); with protein A beads coated with preimmune, anti-PC, anti-BRM, anti-Polybromo, or anti-MOR antibodies (α); or with protein G beads coated with anti-OSA antibodies. Following coimmunoprecipitation (IP), samples were resolved by SDS-PAGE, followed by Western blotting to determine whether BRM, Polybromo, MOR, OSA, or the second largest subunit of RNA Pol II (IIc; 140 kDa) was present. (B) The presence of BRM or RNA Pol II in the indicated Bioscale Q10 column fractions was determined by immunoblotting. The chromatographic purification scheme is outlined in Fig. 1A. It should be noted that the majority of RNA Pol II already has been separated from BRM on the Sephacryl S-300 column (data not shown). The majority of BRM eluted from the Bioscale Q10 column at about 240 mM KCl, whereas RNA Pol II eluted at about 450 mM KCl. We failed to detect any overlap in theirelution profiles. (C) OSA and Polybromo form part of two distinct BRM chromatin-remodeling complexes. Drosophila embryo nuclear extracts, concentrated by step elution from a POROS-heparin column (H0.4 fraction), were incubated with protein A beads coated with either affinity-purified rabbit polyclonal antibodies directed against Polybromo or monoclonal antibodies directed against OSA. Proteins retained on the beads after extensive washes with a buffer containing 800 mM KCl and 0.1% NP-40 were resolved by SDS-PAGE on 8% polyacrylamide gels and stained with silver stain. Open triangles indicate the presence of OSA in the anti-OSA immunoprecipitate (BAP complex) and the presence of Polybromo and BAP170 in the anti-Polybromo immunoprecipitate (PBAP complex). The other BRM-associated proteins are components of both the BAP and the PBAP complexes. OSA*, OSA breakdown products. The input, the unbound material, and the beads (mock IP) in the immunoprecipitation reactions are shown. IgG, immunoglobulin G. (D) BAP170 is found solely in the Polybromo-containing complex. Drosophila embryo nuclear extracts were incubated with protein A beads coated with antibodies directed against BAP170, Polybromo, OSA, or MOR. The immunoprecipitates were resolved by SDS-PAGE, followed by Western blotting with antibodies directed against BAP170, Polybromo, OSA, or MOR.
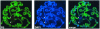
Polybromo is found predominantly in regions of open chromatin on salivary gland polytene chromosomes. The distribution of Polybromo on wild-type polytene chromosomes was determined by indirect immunofluorescence with affinity-purified polyclonal antibodies (green) directed against the protein. DNA was visualized by DAPI staining (blue). The arrowheads indicate the inactive chromocenter and chromosome 4. (Left panel) Indirect immunofluorescence with an anti-Polybromo (PB) antibody (green). (Middle panel) DAPI-stained DNA (blue). (Right panel) Merge image revealing the predominant localization of Polybromo at the interband regions of open chromatin, which are stained weakly with DAPI.
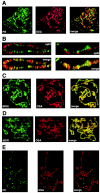
Polybromo and OSA display distinct but overlapping patterns of distribution on polytene chromosomes. The distributions of Polybromo, OSA, BRM, MOR, and PC on wild-type polytene chromosomes were determined by indirect immunofluorescence with affinity-purified polyclonal antibodies directed against Polybromo, BRM, MOR, and PC (all green) and monoclonal antibodies directed against OSA (red). (A) Localization of Polybromo (PB) (green) and OSA (red). (B) Higher magnifications of two areas of panel A. The split and merge images illustrate the distinct patterns of distribution of OSA and Polybromo. (C) Localization of BRM (green) and OSA (red). (D) Localization of MOR (green) and OSA (red). (E) Localization of PC (green) and OSA (red).
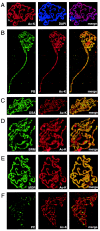
The BRM chromatin-remodeling complexes, but not the PC repressor, associate with regions of hyperacetylated chromatin. (A) Regions of open chromatin are hyperacetylated. The distribution of acetyllysine residues (Ac-K) on polytene chromosomes was determined by indirect immunofluorescence with a sheep polyclonal antibody (red). DNA was visualized by DAPI staining (blue). The arrowhead indicates the inactive chromocenter. The merge image reveals the predominant localization of Ac-K at the interband regions, which are stained weakly with DAPI. (B to E) Colocalization of Polybromo (PB)(B), OSA (C), BRM (D), and MOR (E) (all green) with hyperacetylated chromatin (Ac-K) (red). (F) PC is associated with regions that are not acetylated. PC (green) binds hypoacetylated chromatin, which is stained weakly with antibodies directed against Ac-K (red).
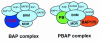
Drosophila contains two distinct BRM complexes: BAP and PBAP. To determine the relationship between the BRM complex and the SWI/SNF-BAF or RSC-PBAF subclass of remodelers, we purified BRM and its associated factors and identified two novel subunits: Drosophila Polybromo and BAP170. These findings in turn allowed us to define two distinct Drosophila BRM chromatin-remodeling complexes: BAP and PBAP. The former is the Drosophila counterpart of SWI/SNF-BAF, and the latter corresponds to RSC-PBAF. BAP and PBAP share seven identical subunits (light blue), which include the central ATPase BRM, trxG protein MOR, actin, and an actin-like protein (BAP55). The distinguishing subunits are OSA in the BAP complex and Polybromo (PB) and BAP170 in the PBAP complex. Determination of the genome-wide distributions of OSA and Polybromo on larval salivary gland polytene chromosomes revealed differential targeting of BAP and PBAP. These results suggest an evolutionarily conserved structural and functional differentiation between the SWI/SNF-BAF-BAP and the RSC-PBAF-PBAP subfamilies of remodelers.
Similar articles
-
Chalkley GE, Moshkin YM, Langenberg K, Bezstarosti K, Blastyak A, Gyurkovics H, Demmers JA, Verrijzer CP. Chalkley GE, et al. Mol Cell Biol. 2008 May;28(9):2920-9. doi: 10.1128/MCB.02217-07. Epub 2008 Feb 25. Mol Cell Biol. 2008. PMID: 18299390 Free PMC article.
-
Functional differentiation of SWI/SNF remodelers in transcription and cell cycle control.
Moshkin YM, Mohrmann L, van Ijcken WF, Verrijzer CP. Moshkin YM, et al. Mol Cell Biol. 2007 Jan;27(2):651-61. doi: 10.1128/MCB.01257-06. Epub 2006 Nov 13. Mol Cell Biol. 2007. PMID: 17101803 Free PMC article.
-
He J, Xuan T, Xin T, An H, Wang J, Zhao G, Li M. He J, et al. PLoS One. 2014 Jul 28;9(7):e103473. doi: 10.1371/journal.pone.0103473. eCollection 2014. PLoS One. 2014. PMID: 25068272 Free PMC article.
-
The SWI/SNF family of ATP-dependent chromatin remodelers: similar mechanisms for diverse functions.
Wang W. Wang W. Curr Top Microbiol Immunol. 2003;274:143-69. doi: 10.1007/978-3-642-55747-7_6. Curr Top Microbiol Immunol. 2003. PMID: 12596907 Review.
-
The Many Roles of BAF (mSWI/SNF) and PBAF Complexes in Cancer.
Hodges C, Kirkland JG, Crabtree GR. Hodges C, et al. Cold Spring Harb Perspect Med. 2016 Aug 1;6(8):a026930. doi: 10.1101/cshperspect.a026930. Cold Spring Harb Perspect Med. 2016. PMID: 27413115 Free PMC article. Review.
Cited by
-
Duan Y, Tian L, Gao Q, Liang L, Zhang W, Yang Y, Zheng Y, Pan E, Li S, Tang N. Duan Y, et al. Oncotarget. 2016 Jul 19;7(29):45863-45875. doi: 10.18632/oncotarget.10244. Oncotarget. 2016. PMID: 27351279 Free PMC article.
-
Epigenetic inheritance and gene expression regulation in early Drosophila embryos.
Ciabrelli F, Atinbayeva N, Pane A, Iovino N. Ciabrelli F, et al. EMBO Rep. 2024 Oct;25(10):4131-4152. doi: 10.1038/s44319-024-00245-z. Epub 2024 Sep 16. EMBO Rep. 2024. PMID: 39285248 Free PMC article. Review.
-
Bracken AP, Brien GL, Verrijzer CP. Bracken AP, et al. Genes Dev. 2019 Aug 1;33(15-16):936-959. doi: 10.1101/gad.326066.119. Epub 2019 May 23. Genes Dev. 2019. PMID: 31123059 Free PMC article. Review.
-
Chalkley GE, Moshkin YM, Langenberg K, Bezstarosti K, Blastyak A, Gyurkovics H, Demmers JA, Verrijzer CP. Chalkley GE, et al. Mol Cell Biol. 2008 May;28(9):2920-9. doi: 10.1128/MCB.02217-07. Epub 2008 Feb 25. Mol Cell Biol. 2008. PMID: 18299390 Free PMC article.
-
Tian Y, Smith-Bolton RK. Tian Y, et al. Genetics. 2021 Mar 3;217(1):1-16. doi: 10.1093/genetics/iyaa028. Genetics. 2021. PMID: 33683366 Free PMC article.
References
-
- Becker, P. B., and W. Horz. 2002. ATP-dependent nucleosome remodeling. Annu. Rev. Biochem. 71:247-273. - PubMed
-
- Beisel, C., A. Imhof, J. Greene, E. Kremmer, and F. Sauer. 2002. Histone methylation by the Drosophila epigenetic transcriptional regulator Ash1. Nature 419:857-862. - PubMed
-
- Boyer, L. A., C. Logie, E. Bonte, P. B. Becker, P. A. Wade, A. P. Wolffe, C. Wu, A. N. Imbalzano, and C. L. Peterson. 2000. Functional delineation of three groups of the ATP-dependent family of chromatin remodeling enzymes. J. Biol. Chem. 275:18864-18870. - PubMed
-
- Cairns, B. R., H. Erdjument-Bromage, P. Tempst, F. Winston, and R. D. Kornberg. 1998. Two actin-related proteins are shared functional components of the chromatin-remodeling complexes RSC and SWI/SNF. Mol. Cell 2:639-651. - PubMed
Publication types
MeSH terms
Substances
LinkOut - more resources
Full Text Sources
Molecular Biology Databases
Miscellaneous