Migration of perilesional microglia after focal brain injury and modulation by CC chemokine receptor 5: an in situ time-lapse confocal imaging study - PubMed
- ️Sat Jan 01 2005
Migration of perilesional microglia after focal brain injury and modulation by CC chemokine receptor 5: an in situ time-lapse confocal imaging study
W Shawn Carbonell et al. J Neurosci. 2005.
Erratum in
- J Neurosci. 2005 Aug 10;25(32):table of contents
Abstract
Microglia rapidly become reactive in response to diverse stimuli and are thought to be prominent participants in the pathophysiology of both acute injury and chronic neurological diseases. However, mature microglial reactions to a focal lesion have not been characterized dynamically in adult vertebrate tissue. Here, we present a detailed analysis of long-distance perilesional microglial migration using time-lapse confocal microscopy in acutely isolated living slices from adult brain-injured mice. Extensive migration of perilesional microglia was apparent by 24 h after injury and peaked at 3 d. Average instantaneous migration speeds of approximately 5 microm/min and peak speeds >10 microm/min were observed. Collective, directed migration toward the lesion edge was not observed as might be expected in the presence of chemoattractive gradients. Rather, migration was autonomous and could be modeled as a random walk. Pharmacological blockade of the cysteine-cysteine chemokine receptor 5 reduced migration velocity and the number of perilesional migratory microglia without affecting directional persistence, suggesting a novel role for chemokines in modulation of discrete migratory parameters. Finally, activated microglia in the denervated hippocampal stratum oriens did not migrate extensively, whereas human immunodeficiency virus-1 tat-activated microglia migrated nearly twice as fast as those at the stab lesion, indicating a nonuniform microglial response to different stimuli. Understanding the characteristics and specific molecular mechanisms underlying microglial migration after neural injury could reveal novel targets for therapeutic strategies for modulating neuroinflammation in human diseases.
Figures
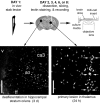
Procedures for confocal time-lapse videomicrography of microglia after focal lesion. Adult mice were subjected to forebrain stab lesion on the first day. One to 7 d after injury, the brains were rapidly collected, sliced coronally at 300 μm with a vibratome, and stained with fluorescently tagged IB4 lectin to visualize microglia/macrophages. Confocal recording was performed for 1-4 h in the regions denoted by the black stars. Microglia become activated in response to both indirect and direct damage produced by forebrain stab lesion. Bottom right, Activated microglia visualized with IB4 lectin at the stab lesion are widespread in the ipsilateral thalamus (white arrows; stab lesion). Scale bar, 50 μm. Bottom left, Activated microglia responding to deafferentation can be found in the stratum oriens of the hippocampus in the contralateral hemisphere by 3 d. Scale bar, 100 μm. ca3, Stratum pyramidale of the hippocampus; ff, ventromedial fimbria/fornix; so, stratum oriens of the hippocampus; v, lateral ventricle.
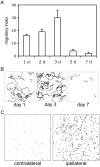
Characterization of perilesional migration. A, The peak of cellular migration occurs at day 3 with substantial decline by day 7. The migratory index represents the mean migratory cell number per 75 × 75 μm subfield. See movies 1-5 (available at
www.jneurosci.orgas
supplemental material). B, Representative manual cell path tracings of migratory microglia from quantitated subfields at 1, 3, and 7 d after lesion. C, Cellular migration is not caused by the brain slicing procedure. Automated cell track tracings were performed from slices vitally stained with Syto 16 for nuclei in 300 × 300 μm fields sampled from the uninjured (left) or injured (right) thalamus 24 h after lesion (fields are from the identical slice; n = 3 independent experiments). Migratory cells were only found in the injured hemisphere, demonstrating that the slicing procedure itself did not acutely stimulate migration. See movie 6 (available at
www.jneurosci.orgas
supplemental material).
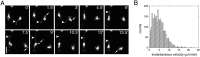
In situ motility of perilesional microglia. A, Migratory microglia demonstrated transient forward protrusions (white arrows) and long-lasting trailing processes (white arrowheads). Scale bar, 30 μm. B, Distribution of lateral velocities of continuously migrating microglia measured at 90 s intervals (n = 2815 measurements from 47 cells; 4 slices from 4 different mice). Each frame in A is a two-dimensional projection representing a stack of six images 8 μm apart (40 μm depth).
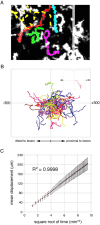
Random walk perilesional microglial migration. A, Perilesional microglial migration is not collective nor highly directed (movie 11, available at
www.jneurosci.orgas
supplemental material). B, Normalized cell paths of continuously migrating microglia plotted from a common origin demonstrates migration of microglia in all directions with no obvious evidence for directional bulk flow (n = 47 cells; 4 slices from 4 different mice). The mean (inset) and median (data not shown) paths demonstrate only a minor bias toward the lesion. Units are in micrometers. C, The mean absolute displacement of continuously migrating microglia plotted as a function of the square root of time. The close fit to a straight line on this transformed scale demonstrates that migration proceeds essentially as a random walk (R2 = 0.9998).
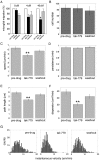
CCR5 modulates microglial migration. Individual slices were recorded sequentially in the absence of drug, in the presence of the highly selective CCR5 inhibitor TAK-779, and after 24 h of drug washout (n = 3 slices from 3 different mice; each dose). A, TAK-779 treatment at 40 μ
mreversibly reduced the number of migratory microglial cells as reflected by percentage of migratory microglia (*p < 0.05). B, Cell viability with 40 μ
mTAK-779 did not differ between the three recording phases as demonstrated by cell number per recording field (p = 0.89). PRW analysis was applied to the 40 μ
mTAK-779 experiments revealing significant (**p < 0.01) differences between predrug conditions and TAK-779 for cell speed (C), path length (E), and dispersion (F). D, There were no differences in directional persistence times. All parameters could be rescued to near pretreatment values after drug washout. G, TAK-779 treatment (40 μ
m) resulted in a leftward shift of the distribution histogram of instantaneous velocities (mean, 4.57 ± 0.11 μm/min) compared with pretreatment (mean, 6.65 ± 0.15 μm/min). Drug washout (mean, 6.30 ± 0.15 μm/min) rescued velocity distribution to near-baseline conditions.
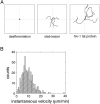
Microglial migration responses are stimulus dependent. A, Trajectories of five representative migrating cells for each condition were traced for a 15 min recording period and plotted from a common origin. Microglia migrated extensively in the perilesional thalamus at 3 d (middle) but not in the contralateral hippocampus at the site of denervation at 3 d (left). Microglia activated by HIV-1 tat protein migrated the greatest distances. Plots measure, 150 × 150 μm. B, Microglia activated by an intracerebroventricular injection of HIV-1 tat protein 24 h before slice collection migrated approximately twice as fast as cells in the perilesional thalamus at 24 h (compare with Fig. 3B) (see movie 14, available at
www.jneurosci.orgas
supplemental material).
Similar articles
-
Kurpius D, Nolley EP, Dailey ME. Kurpius D, et al. Glia. 2007 Jun;55(8):873-84. doi: 10.1002/glia.20509. Glia. 2007. PMID: 17405148
-
Louboutin JP, Strayer DS. Louboutin JP, et al. CNS Neurol Disord Drug Targets. 2013 Sep;12(6):815-29. doi: 10.2174/18715273113126660173. CNS Neurol Disord Drug Targets. 2013. PMID: 24047524 Review.
-
Grossmann R, Stence N, Carr J, Fuller L, Waite M, Dailey ME. Grossmann R, et al. Glia. 2002 Mar 1;37(3):229-40. Glia. 2002. PMID: 11857681
-
Ex vivo dynamic imaging of retinal microglia using time-lapse confocal microscopy.
Lee JE, Liang KJ, Fariss RN, Wong WT. Lee JE, et al. Invest Ophthalmol Vis Sci. 2008 Sep;49(9):4169-76. doi: 10.1167/iovs.08-2076. Epub 2008 May 16. Invest Ophthalmol Vis Sci. 2008. PMID: 18487378 Free PMC article.
-
Microglia in the TBI brain: The good, the bad, and the dysregulated.
Loane DJ, Kumar A. Loane DJ, et al. Exp Neurol. 2016 Jan;275 Pt 3(0 3):316-327. doi: 10.1016/j.expneurol.2015.08.018. Epub 2015 Sep 3. Exp Neurol. 2016. PMID: 26342753 Free PMC article. Review.
Cited by
-
Bou Sader Nehme S, Sanchez-Sarasua S, Adel R, Tuifua M, Ali A, Essawy AE, Abdel Salam S, Hleihel W, Boué-Grabot E, Landry M. Bou Sader Nehme S, et al. Front Pharmacol. 2024 Jan 4;14:1288994. doi: 10.3389/fphar.2023.1288994. eCollection 2023. Front Pharmacol. 2024. PMID: 38239187 Free PMC article.
-
Guenther C. Guenther C. Front Immunol. 2022 Apr 22;13:809590. doi: 10.3389/fimmu.2022.809590. eCollection 2022. Front Immunol. 2022. PMID: 35529883 Free PMC article. Review.
-
Sabate-Soler S, Nickels SL, Saraiva C, Berger E, Dubonyte U, Barmpa K, Lan YJ, Kouno T, Jarazo J, Robertson G, Sharif J, Koseki H, Thome C, Shin JW, Cowley SA, Schwamborn JC. Sabate-Soler S, et al. Glia. 2022 Jul;70(7):1267-1288. doi: 10.1002/glia.24167. Epub 2022 Mar 9. Glia. 2022. PMID: 35262217 Free PMC article.
-
Targeting Members of the Chemokine Family as a Novel Approach to Treating Neuropathic Pain.
Pawlik K, Mika J. Pawlik K, et al. Molecules. 2023 Jul 30;28(15):5766. doi: 10.3390/molecules28155766. Molecules. 2023. PMID: 37570736 Free PMC article. Review.
-
CXCR4 involvement in neurodegenerative diseases.
Bonham LW, Karch CM, Fan CC, Tan C, Geier EG, Wang Y, Wen N, Broce IJ, Li Y, Barkovich MJ, Ferrari R, Hardy J, Momeni P, Höglinger G, Müller U, Hess CP, Sugrue LP, Dillon WP, Schellenberg GD, Miller BL, Andreassen OA, Dale AM, Barkovich AJ, Yokoyama JS, Desikan RS; International FTD-Genomics Consortium (IFGC); International Parkinson’s Disease Genetics Consortium (IPDGC); International Genomics of Alzheimer’s Project (IGAP). Bonham LW, et al. Transl Psychiatry. 2018 Apr 11;8(1):73. doi: 10.1038/s41398-017-0049-7. Transl Psychiatry. 2018. PMID: 29636460 Free PMC article.
References
-
- Bajetto A, Bonavia R, Barbero S, Schettini G (2002) Characterization of chemokines and their receptors in the central nervous system: physiopathological implications. J Neurochem 82: 1311-1329. - PubMed
Publication types
MeSH terms
Substances
Grants and funding
LinkOut - more resources
Full Text Sources
Other Literature Sources