Dominant-negative mutant phenotypes and the regulation of translation elongation factor 2 levels in yeast - PubMed
- ️Sat Jan 01 2005
Dominant-negative mutant phenotypes and the regulation of translation elongation factor 2 levels in yeast
Pedro A Ortiz et al. Nucleic Acids Res. 2005.
Abstract
The eukaryotic translation elongation factor 2 (eEF2), a member of the G-protein superfamily, catalyzes the post-peptidyl transferase translocation of deacylated tRNA and peptidyl tRNA to the ribosomal E- and P-sites. eEF2 is modified by a unique post-translational modification: the conversion of His699 to diphthamide at the tip of domain IV, the region proposed to mimic the anticodon of tRNA. Structural models indicate a hinge is important for conformational changes in eEF2. Mutations of V488 in the hinge region and H699 in the tip of domain IV produce non-functional mutants that when co-expressed with the wild-type eEF2 result in a dominant-negative growth phenotype in the yeast Saccharomyces cerevisiae. This phenotype is linked to reduced levels of the wild-type protein, as total eEF2 levels are unchanged. Changes in the promoter, 5'-untranslated region (5'-UTR) or 3'-UTR of the EFT2 gene encoding eEF2 do not allow overexpression of the protein, showing that eEF2 levels are tightly regulated. The H699K mutant, however, also alters translation phenotypes. The observed regulation suggests that the cell needs an optimum amount of active eEF2 to grow properly. This provides information about a new mechanism by which translation is efficiently maintained.
Figures
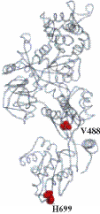
Structure of yeast eEF2 indicates the location of V488 in the polylinker region and H699 at the tip of domain IV. The structure was produced with the PyMOL program (37), using PDB 1NOV coordinates (8).
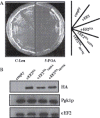
eEF2V499A and eEF2H699K are unable to function as the only form of eEF2 in vivo and confer a dominant-negative phenotype. (A) YEFD12h transformed with pRS315 (empty), pTKB615 (eEF2), pTKB501 (eEF2HA), pTKB696 (eEF2V488AHA) and pTKB701 (eEF2H699KHA) were streaked on C-Leu or 5-FOA and incubated from 3 to 7 days at 30°C. (B) Western blot analysis against the HA tag shows that the mutant proteins are expressed. Lanes are empty vector, eEF2HA, eEF2V488AHA and eEF2H699KHA. Protein extracts were prepared, resolved by SDS–PAGE, and detected with antibodies against HA, eEF2 and Pgk1p (loading control).
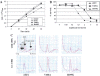
Strains expressing eEF2H699K affect translation at the elongation step. (A) Strain YEFD12h expressing no additional copies of eEF2 (pRS315, crosses), eEF2 (pTKB612, diamonds), eEF2V488A (pTKB658, squares) or eEF2H699K (pTKB704, triangles) were grown in C-Met-Leu to mid-log phase. [35S]methionine was added and total protein synthesis was measured at each time point by TCA precipitation. (B) eEF2H699K confers dominant sensitivity to paromomycin. YEFD12h transformed with eEF2 (pTKB501, diamonds), eEF2V488A (pTKB696, squares) or eEF2H699K (pTKB701, triangles) were grown to mid-log phase in C-Leu medium, diluted to A600 = 0.1 in C-Leu in the presence of various concentrations of paromomycin (in triplicate) in 96-well microtiter plates, and grown at 30°C with constant shaking. Growth was monitored by A600 on a Bio-Tek Elx microtiter plate reader and expressed as percent survival relative to untreated cells. (C) Polyribosome analysis shows eEF2H699K confers a defect in translation elongation. Cells were grown at 30°C to mid-log phase, harvested in the presence (with CHX) or absence (w/o CHX) of cycloheximide and extracts were prepared and layered on a 7–47% sucrose gradient. Gradients were centrifuged, fractionated and the A254 monitored.
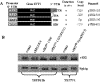
eEF2 protein levels are not controlled by the EFT2 promoter, 5′- or 3′-UTR. (A) Cartoon of eEF2 constructs on low (CEN) and high (2μ) copy number plasmids with EFT2 or TEF5 promoters and 5′-UTR or the EFT2 3′-UTR is replaced with a 6× His or HA tag. (B) Plasmids expressing empty vector, eEF2 CEN (EFT2 promoter), eEF2HA CEN (EFT2 promoter) and eEF2HIS CEN (TEF5 promoter) were transformed into YEFD12h, and eEF2HIS 2µ (TEF5 promoter) was transformed into TKY751. Protein extracts were prepared, resolved by SDS–PAGE, and detected with antibodies against eEF2 and Pgk1p (loading control). eEF2 expression is expressed relative to Pgk1p levels.
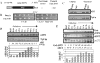
Transient overexpression of eEF2 demonstrates protein levels are reduced before mRNA levels. (A) Construct in which eEF2 is expressed under the GAL1 galactose inducible promoter. (B) YEFD12h was transformed with pRS315 (empty) and pTKB763 (GAL1 eEF2HIS). Cells were grown to mid-log phase at 30°C in C-Leu liquid media then spotted as 10-fold serial dilutions on C-Leu and C-Leu plus galactose. (C) Protein extracts were prepared from the strains as in (B), grown to steady state in the absence and presence of galactose. Total proteins were resolved by SDS–PAGE and detected with antibodies against eEF2 and Pgk1p. The fold expression is calculated relative to Pgk1p levels. (D) YEFD12h with pTKB763 (GAL1 eEF2HIS) was grown to mid-log phase in C-Leu plus raffinose media and a sample taken. The culture was harvested, washed with water and resuspended in C-Leu plus galactose. Subsequently samples were taken for each time point, protein extracts prepared, resolved by SDS–PAGE and detected with antibodies against eEF2 and Pgk1p. The numbers indicate eEF2 expression relative to Pgk1p levels. The bar graph shows eEF2 expression levels at each galactose time point relative to expression in raffinose media. (E) RNA extracts were prepared from the same time points as (D), resolved by a formaldehyde agarose gel and detected with probes against EFT2 and ACT1 (loading control). The numbers indicate mRNA expression from each eEF2-expressing plasmid relative to ACT1. The bar graph shows total EFT2 mRNA expression relative to ACT1 mRNA.
Similar articles
-
Ortiz PA, Ulloque R, Kihara GK, Zheng H, Kinzy TG. Ortiz PA, et al. J Biol Chem. 2006 Oct 27;281(43):32639-48. doi: 10.1074/jbc.M607076200. Epub 2006 Sep 1. J Biol Chem. 2006. PMID: 16950777
-
Tight interaction of eEF2 in the presence of Stm1 on ribosome.
Hayashi H, Nagai R, Abe T, Wada M, Ito K, Takeuchi-Tomita N. Hayashi H, et al. J Biochem. 2018 Mar 1;163(3):177-185. doi: 10.1093/jb/mvx070. J Biochem. 2018. PMID: 29069440
-
Chaperone Function of Hgh1 in the Biogenesis of Eukaryotic Elongation Factor 2.
Mönkemeyer L, Klaips CL, Balchin D, Körner R, Hartl FU, Bracher A. Mönkemeyer L, et al. Mol Cell. 2019 Apr 4;74(1):88-100.e9. doi: 10.1016/j.molcel.2019.01.034. Epub 2019 Mar 12. Mol Cell. 2019. PMID: 30876804
-
Eukaryotic elongation factor-2 (eEF2): its regulation and peptide chain elongation.
Kaul G, Pattan G, Rafeequi T. Kaul G, et al. Cell Biochem Funct. 2011 Apr;29(3):227-34. doi: 10.1002/cbf.1740. Epub 2011 Mar 10. Cell Biochem Funct. 2011. PMID: 21394738 Review.
-
SSD1 modifies phenotypes of Elongator mutants.
Xu F, Byström AS, Johansson MJO. Xu F, et al. Curr Genet. 2020 Jun;66(3):481-485. doi: 10.1007/s00294-019-01048-9. Epub 2019 Nov 27. Curr Genet. 2020. PMID: 31776648 Free PMC article. Review.
Cited by
-
Spinocerebellar ataxias (SCAs) caused by common mutations.
Müller U. Müller U. Neurogenetics. 2021 Oct;22(4):235-250. doi: 10.1007/s10048-021-00662-5. Epub 2021 Aug 16. Neurogenetics. 2021. PMID: 34401960 Free PMC article. Review.
-
Pseudomonas Exotoxin A: optimized by evolution for effective killing.
Michalska M, Wolf P. Michalska M, et al. Front Microbiol. 2015 Sep 15;6:963. doi: 10.3389/fmicb.2015.00963. eCollection 2015. Front Microbiol. 2015. PMID: 26441897 Free PMC article. Review.
-
Hawer H, Ütkür K, Arend M, Mayer K, Adrian L, Brinkmann U, Schaffrath R. Hawer H, et al. PLoS One. 2018 Oct 18;13(10):e0205870. doi: 10.1371/journal.pone.0205870. eCollection 2018. PLoS One. 2018. PMID: 30335802 Free PMC article.
-
Mallory MJ, McClory SP, Chatrikhi R, Gazzara MR, Ontiveros RJ, Lynch KW. Mallory MJ, et al. Nucleic Acids Res. 2020 Jun 4;48(10):5710-5719. doi: 10.1093/nar/gkaa295. Nucleic Acids Res. 2020. PMID: 32338744 Free PMC article.
-
Functional features of the C-terminal region of yeast ribosomal protein L5.
Moradi H, Simoff I, Bartish G, Nygård O. Moradi H, et al. Mol Genet Genomics. 2008 Oct;280(4):337-50. doi: 10.1007/s00438-008-0369-7. Epub 2008 Aug 27. Mol Genet Genomics. 2008. PMID: 18751732
References
-
- Hershey J.W.B., Merrick W.C. Pathway and Mechanism of Initiation of Protein Synthesis. In: Sonenberg N., Hershey J.W.B., Mathews M.B., editors. Translational Control of Gene Expression. Cold Spring Harbor: Cold Spring Harbor Laboratory Press; 2000. pp. 33–88.
-
- Merrick W.C., Nyborg J. The Protein Biosynthesis Elongation Cycle. In: Sonenberg N., Hershey J.W.B., Mathews M.B., editors. Translational Control of Gene Expression. Cold Spring Harbor: Cold Spring Harbor Laboratory; 2000. pp. 89–126.
-
- Welch E.M., Wang W., Peltz S.W. Translation Termination: It's Not the End of the Story. In: Sonenberg N., Hershey J.W.B., Mathews M.B., editors. Translational Control of Gene Expression. Cold Spring Harbor: Cold Spring Harbor Laboratory; 2000. pp. 467–485.
-
- Proud C.G. Regulation of mRNA translation. Essays Biochem. 2001;37:97–108. - PubMed
-
- Huang Y.S., Richter J.D. Regulation of local mRNA translation. Curr. Opin. Cell Biol. 2004;16:308–313. - PubMed
Publication types
MeSH terms
Substances
LinkOut - more resources
Full Text Sources
Molecular Biology Databases
Miscellaneous