Neuronal responses to transient hypoglycaemia in the dorsal vagal complex of the rat brainstem - PubMed
- ️Sun Jan 01 2006
Neuronal responses to transient hypoglycaemia in the dorsal vagal complex of the rat brainstem
Robert H Balfour et al. J Physiol. 2006.
Abstract
Several regions of the mammalian brain contain glucosensing neurones. In vivo studies have suggested that those located in the hypothalamus and lower brainstem are involved in glucoprivic feeding and homeostatic control of blood glucose. We have identified and characterized hypoglycaemia-sensitive neurones in the dorsal vagal complex of the brainstem using in situ hybridization, single-cell RT-PCR and whole-cell patch-clamp recordings from rat brainstem slices. Approximately 80% of neurones did not respond to hypoglycaemia (changing artificial cerebrospinal fluid (ACSF) glucose from 10 mM to 0 mM) within 5 min (non-responsive: NR). Another 10% depolarized within 155+/-31 s (mean+/-s.e.m.) of glucose removal (glucose-inhibited: GI), and the remaining neurones hyperpolarized within 53+/-7 s (glucose-excited: GE). The hyperpolarization was reversed by the KATP channel blocker tolbutamide. Single-cell RT-PCR revealed that GI and GE, but not NR, cells expressed glucokinase (GLK). In contrast, SUR1, a KATP channel subunit, was expressed in GE and some NR cells. In situ hybridization with biotin-labelled riboprobes in the dorsal vagal complex revealed ubiquitous expression of SUR1, and widespread, but sparse, expression of GLK. Identification of astrocytes using a GFAP (glial fibrillary acidic protein) antibody showed that GLK and GFAP were not colocalized. In summary, we have demonstrated that GI and GE neurones exist in the brainstem and that GLK is essential for their function. It seems likely that GE neurones work in a way analogous to pancreatic beta-cells in that they require both GLK and KATP channels.
Figures
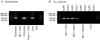
A, 2% agarose gel demonstrating the 525 bp PCR product for glucokinase obtained with the primers specified in Table 1 from cDNA generated from brainstem, pancreas and liver mRNA. BS 1: 1000: brainstem cDNA diluted 1000 ×; Pancr: pancreas cDNA; H2O: negative control using deionized water instead of cDNA. B, 2% agarose gel demonstrating the sensitivity of primers for KATP channel subunits SUR1 (493 bp PCR product) and Kir6.2 (478 bp PCR product). Both subunits could be amplified from brainstem cDNA in a 10 000 ×, but not 100 000 ×, dilution.
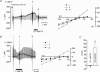
A, left, voltage-clamp recording demonstrating spontaneous outward current triggered by whole-cell configuration (ATP-free pipette solution). Tolbutamide was bath applied as indicated by the black bar. Right, current–voltage relations for time points indicated. B, voltage-clamp trace from a DVN. Downward deflections indicate the current response to −40 mV voltage steps of 500 ms duration every 5 s. i, ii and iii indicate current responses to voltage pulses used to generate current–voltage relations shown. C, mean change in holding current at −60 mV upon application of 0.1 m
mtolbutamide (TB) or 0.2 m
mdiazoxide (DZ).
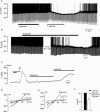
A, current-clamp recording from a NR NNST with functional KATP channels as indicated by the response to cyanide. 0 glucose and 1 m
mcyanide (CN) were bath-applied as indicated by the black and grey bars, respectively. Downward deflections of the voltage trace were caused by alternating current pulses of −60 and −80 pA amplitude and 500 ms duration every 5 s. Action potential amplitude is truncated at −20 mV. B, prolonged exposure (23 min) of an NR neurone to glucose-free solution as indicated by the black bar led to a reversible hyperpolarization and increase in membrane conductance. Downward deflections represent the voltage response to repetitive 500 ms injections of −60 pA current. C, voltage-clamp recording from an NR neurone. 5 min of 0 m
mand 30 min of 2 m
mglucose had no clear effect on the standing outward current at −20 mV. In contrast, 3 m
msodium azide or prolonged exposure to glucose-free ACSF led to a reversible, pronounced increase in outward current. D, current–voltage relations from the recording in C at various time points. Prolonged exposure to zero glucose, but not 2 m
mglucose evoked a current with a reversal potential near EK that is abolished by 0.1 m
mtolbutamide (E). F, mean time to onset of the hypoglycaemia-induced current with and without preincubation in 2 m
mglucose. TB: tolbutamide; CN: sodium cyanide; AZ: sodium azide. **P < 0.01 unpaired t test.
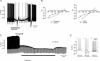
Current clamp recordings from GE neurones. A, fast and reversible hyperpolarization of a DVN upon glucose removal. B, whole-cell current–voltage relations from a DVN in the presence of 10 m
m(control) or 0 m
mglucose or 2-deoxyglucose (2-DG). The differential current (hypoglycaemia-induced) reversed close to EK. C, recording from an NNST demonstrating that the hypoglycaemia-induced hyperpolarization persisted in 0.5 μ
mtetrodotoxin (TTX) and was inhibited by tolbutamide (TB). D, large variations in amplitude of afterhyperpolarization were observed between different GE cells (i, ii, iii represent different cells recorded).
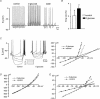
A, current clamp recording from a GI neurone before, during and after exposure to glucose-free ACSF. No holding current and no current pulses were applied. B, mean data from 8 GI cells recorded as in A. **P < 0.01, paired t test. C–E, current–voltage relations from 3 GI cells recorded in 10 and 0 m
mglucose. In response to glucose removal, cells either increased conductance (reversal potential: ∼0 mV (C) or ∼−40 mV (E)) or decreased conductance (reversal potential ∼−90 mV (D)). Reversal potentials were obtained by extrapolating the linear part of the I–V relation (to avoid contributions by voltage-gated channels).
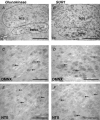
Glucokinase (GLK) mRNA detection by in situ hybridization in the dorsal vagal nucleus (DMNX; A and C) and nucleus tractus solitarius (NTS; A and E), GLK-positive cells (examples indicated by black arrows) showed cytosolic staining with the nucleus spared. Differential interference contrast (DIC) optics (C and E) allowed visualization of unstained (GLK-negative) cells (examples indicated by white arrows). CC, central canal. In situ hybridization for SUR1 within the DMNX (B and D) and NTS (B and F). SUR1-positive cells showed cytosolic staining with the nucleus spared (examples indicated by black arrows. White arrows: negative cells). A and B bright field optics, C–F bright field with DIC. Scale bars: A and B, 100 μm; C–F, 50 μm.
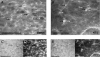
Glucokinase mRNA (detected by in situ hybridization, indicated with white arrows) was not colocalized with GFAP immunoreactivity (indicated by black arrows, showing the characteristic star-like appearance of astrocytes) in the dorsal vagal nucleus (A) or in the nucleus tractus solitarii (B). A and B are composites of the glucokinase signal observed under bright field optics (C and D) and the GFAP fluorescence (E and F). Scale bars: 100 μm.
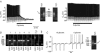
A, left, GE cell that hyperpolarized in response to glucose removal and expressed glucokinase (525 bp product, arrow). Right, NR cell that lacked response to removal of glucose and lacked glucokinase expression. B, typical single-cell RT-PCR analysis for glucokinase (GLK) and SUR1 of 5 neurones. 1, 2, 4, 5: NR neurones; 3: GE neurone. Controls: PIP, pipette solution without cytoplasm extracted from cell. H2O, no cDNA. C, current clamp recording from a GI neurone positive for GLK mRNA.
Similar articles
-
Balfour RH, Trapp S. Balfour RH, et al. J Physiol. 2007 Mar 15;579(Pt 3):691-702. doi: 10.1113/jphysiol.2006.126094. Epub 2007 Jan 11. J Physiol. 2007. PMID: 17218356 Free PMC article.
-
Karschin A, Brockhaus J, Ballanyi K. Karschin A, et al. J Physiol. 1998 Jun 1;509 ( Pt 2)(Pt 2):339-46. doi: 10.1111/j.1469-7793.1998.339bn.x. J Physiol. 1998. PMID: 9575284 Free PMC article.
-
Lee K, Dixon AK, Richardson PJ, Pinnock RD. Lee K, et al. J Physiol. 1999 Mar 1;515 ( Pt 2)(Pt 2):439-52. doi: 10.1111/j.1469-7793.1999.439ac.x. J Physiol. 1999. PMID: 10050011 Free PMC article.
-
Glucose effects on gastric motility and tone evoked from the rat dorsal vagal complex.
Ferreira M Jr, Browning KN, Sahibzada N, Verbalis JG, Gillis RA, Travagli RA. Ferreira M Jr, et al. J Physiol. 2001 Oct 1;536(Pt 1):141-52. doi: 10.1111/j.1469-7793.2001.t01-1-00141.x. J Physiol. 2001. PMID: 11579164 Free PMC article.
-
Dissociation between sensing and metabolism of glucose in sugar sensing neurones.
Gonzàlez JA, Reimann F, Burdakov D. Gonzàlez JA, et al. J Physiol. 2009 Jan 15;587(1):41-8. doi: 10.1113/jphysiol.2008.163410. Epub 2008 Nov 3. J Physiol. 2009. PMID: 18981030 Free PMC article. Review.
Cited by
-
Balfour RH, Trapp S. Balfour RH, et al. J Physiol. 2007 Mar 15;579(Pt 3):691-702. doi: 10.1113/jphysiol.2006.126094. Epub 2007 Jan 11. J Physiol. 2007. PMID: 17218356 Free PMC article.
-
Neural pathways that control the glucose counterregulatory response.
Verberne AJ, Sabetghadam A, Korim WS. Verberne AJ, et al. Front Neurosci. 2014 Feb 26;8:38. doi: 10.3389/fnins.2014.00038. eCollection 2014. Front Neurosci. 2014. PMID: 24616659 Free PMC article. Review.
-
Physiological functions of glucose-inhibited neurones.
Burdakov D, González JA. Burdakov D, et al. Acta Physiol (Oxf). 2009 Jan;195(1):71-8. doi: 10.1111/j.1748-1716.2008.01922.x. Epub 2008 Oct 28. Acta Physiol (Oxf). 2009. PMID: 18983451 Free PMC article.
-
Browning KN, Travagli RA. Browning KN, et al. Compr Physiol. 2014 Oct;4(4):1339-68. doi: 10.1002/cphy.c130055. Compr Physiol. 2014. PMID: 25428846 Free PMC article. Review.
-
Espinoza L, Fedorchak S, Boychuk CR. Espinoza L, et al. Front Physiol. 2021 Mar 11;12:624595. doi: 10.3389/fphys.2021.624595. eCollection 2021. Front Physiol. 2021. PMID: 33776789 Free PMC article. Review.
References
-
- Ashcroft FM, Gribble FM. ATP-sensitive K+ channels and insulin secretion: their role in health and disease. Diabetologia. 1999;42:903–919. - PubMed
-
- Ashford ML, Boden PR, Treherne JM. Glucose-induced excitation of hypothalamic neurones is mediated by ATP-sensitive K+ channels. Pflugers Arch. 1990a;415:479–483. - PubMed
-
- Ballanyi K, Kulik A. Intracellular Ca2+ during metabolic activation of KATP channels in spontaneously active dorsal vagal neurons in medullary slices. Eur J Neurosci. 1998;10:2574–2585. - PubMed
MeSH terms
Substances
LinkOut - more resources
Full Text Sources
Medical
Miscellaneous