Base-stacking and base-pairing contributions into thermal stability of the DNA double helix - PubMed
- ️Sun Jan 01 2006
Base-stacking and base-pairing contributions into thermal stability of the DNA double helix
Peter Yakovchuk et al. Nucleic Acids Res. 2006.
Abstract
Two factors are mainly responsible for the stability of the DNA double helix: base pairing between complementary strands and stacking between adjacent bases. By studying DNA molecules with solitary nicks and gaps we measure temperature and salt dependence of the stacking free energy of the DNA double helix. For the first time, DNA stacking parameters are obtained directly (without extrapolation) for temperatures from below room temperature to close to melting temperature. We also obtain DNA stacking parameters for different salt concentrations ranging from 15 to 100 mM Na+. From stacking parameters of individual contacts, we calculate base-stacking contribution to the stability of A*T- and G*C-containing DNA polymers. We find that temperature and salt dependences of the stacking term fully determine the temperature and the salt dependence of DNA stability parameters. For all temperatures and salt concentrations employed in present study, base-stacking is the main stabilizing factor in the DNA double helix. A*T pairing is always destabilizing and G*C pairing contributes almost no stabilization. Base-stacking interaction dominates not only in the duplex overall stability but also significantly contributes into the dependence of the duplex stability on its sequence.
Figures
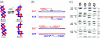
Urea-enhanced gel electrophoresis of nicked and gapped DNA. (a) Nicked DNA molecule undergoes stacked-unstacked transition governed by stacking parameter ΔGKLST. Stacked state is close to intact molecule; unstacked state is approximated by a molecule with a gap. (b) DNA molecules with solitary nicks and gaps in forward (F) or reverse (R) strand were obtained by enzymatic digestion. Nicking enzymes and their recognition sites are shown in one color; cleavage sites are indicated by arrowheads. In KL/F and KL/R fragments nick is positioned within KL/K′L′ dinucleotide stack. Fragments with 2 nt-long gaps, G2/F and G2/R, are the result of sequential digestion by two nicking enzymes as shown. (c) Effect of temperature of PAGE on the separation of intact (I), nicked (N) and gapped (G) DNA fragments. Nicked stacks are shown at the top of each lane; arrowheads point to the location of the nick. Gapped and nicked fragments resolved in one lane have lesions in the same strand. PAGE was conducted in the presence of 2.3 M urea at temperatures indicated to the left of each panel.
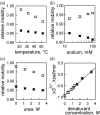
Effect of ambient conditions on PAGE of nicked (open circles) and gapped (closed circles) molecules. Relative mobilities of AA/F and G2/F fragments are measured (a) at different temperatures, PAGE conditions: 1× TBE, 2.3 M urea; (b) as a function of ionic strength, PAGE conditions: 37°C, 3.5 M urea, 1× TBE appended with NaCl (total concentration of sodium assuming 1× TBE to be equivalent to 15 mM Na+ is indicated, see Figure 4); (c) in the presence of urea, PAGE conditions: 37°C, 1× TBE. (d) Effect of denaturant concentration on stacking parameters of AA/F calculated from mobility data using Equations 1 and 2. PAGE was carried out in 1× TBE at 42°C in the presence of varying concentrations of urea (closed diamonds), DMF (open diamonds) or in the absence of the denaturant (dotted diamond).
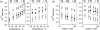
Effect of ambient conditions—temperature (a) and ionic strength (b)—on DNA stacking parameters for A•T- and G•C-containing contacts. Dinucleotide stacks are shown at the top of each panel. Error bars represent the scatter range of the experimentally determined ΔGKLST values of nicked stacks (see text). This data is tabulated in Supplementary Tables 2 and 3.
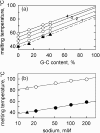
Stability of DNA under different salt conditions. (a) Dependence of TM of 65 bp-long DNA duplexes on their G•C content in 1× TBE (triangles, dashed line) and in 10 mM TE (pH 7.4) (circles, solid lines) appended with NaCl at 20 mM (line 1), 50 mM (line 2), 100 mM (line 3) and 200 mM (line 4). Linear extrapolation to χ = 100% is used to estimated TM of G•C-containing DNA. (b) Stability of A•T-containing (open symbols) and G•C-containing (closed symbols) 65 bp-long duplex DNA as a function of sodium concentration (circles) and in 1×TBE (triangles). TM's of A•T-containing DNA were measured directly; TM's of G•C-containing DNA were estimated by extrapolation shown in (a). For both duplexes, stability in 1× TBE corresponds to the stability in the presence of 15 mM Na+ (as plotted).
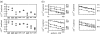
Effect of phosphorylation state of 5′ nt at the nick site on stacked-unstacked equilibrium. (a) Stacking parameters measured in 1× TBE at 37°C of A•T-containing (top panel) and G•C-containing (bottom panel) nicked dinucleotide stacks before (circles) and after (triangles) dephosphorylation are compared. White and gray fills are used for fragments with the nick in the forward and reverse strand, respectively. Nicked dinucleotide stacks with a purine at a 5′-side of the nick are underscored (see text). (b) Salt dependence of ΔGST values of nicked contacts indicated to the right of each panel before (circles) and after (triangles) dephosphorylation. Total concentration of sodium assuming 1× TBE to be equivalent to 15 mM Na+ is indicated, see Figure 4.
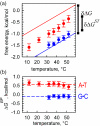
Overall stability, stacking and base pairing contributions for DNA polymers at different temperatures (a) Temperature dependence of the stacking contributions to the stability of A•T- (red circles) and G•C- (blue circles) containing polymers. Straight solid line of the same color gives the temperature dependence of the stability parameter of corresponding polymer calculated using Equations 5 and 6 at [Na+] = 15 mM. (b) Temperature dependence of A•T (red) and G•C (blue) base pairing parameters calculated as a difference between stability and stacking terms using data in (a). Horizontal broken lines correspond to mean values of ΔGA•TBP=0.57 kcal/mol and ΔGG•CBP=−0.11 kcal/mol.
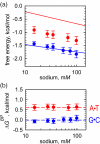
Overall stability, stacking and base pairing contributions for DNA polymers at different salt conditions. (a) Salt dependence of the stacking term in the stability of A•T- (red circles) and G•C- (blue circles) containing polymers. Straight solid lines of the same color correspond to the salt dependence of the DNA polymer stability calculated using Equations 5 and 6 at 37°C. (b) Salt dependence of the base pairing parameters for A•T (red) and G•C (blue) pairs calculated at each concentration as a difference between stability term and stacking term using data from (a). Horizontal broken lines correspond to mean values of ΔGA•TBP=0.61kcal/mol and ΔGG•CBP=−0.01kcal/mol.
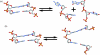
Schematic representation of (a) helix-to-coil transition of a DNA dinucleotide stack and (b) stacked-unstacked equilibrium at a nick site.
Similar articles
-
Stacked-unstacked equilibrium at the nick site of DNA.
Protozanova E, Yakovchuk P, Frank-Kamenetskii MD. Protozanova E, et al. J Mol Biol. 2004 Sep 17;342(3):775-85. doi: 10.1016/j.jmb.2004.07.075. J Mol Biol. 2004. PMID: 15342236
-
DNA melting and energetics of the double helix.
Vologodskii A, Frank-Kamenetskii MD. Vologodskii A, et al. Phys Life Rev. 2018 Aug;25:1-21. doi: 10.1016/j.plrev.2017.11.012. Epub 2017 Nov 14. Phys Life Rev. 2018. PMID: 29170011 Review.
-
Shchyolkina AK, Borisova OF, Livshits MA, Pozmogova GE, Chernov BK, Klement R, Jovin TM. Shchyolkina AK, et al. Biochemistry. 2000 Aug 22;39(33):10034-44. doi: 10.1021/bi9913909. Biochemistry. 2000. PMID: 10955991
-
Counterion association with native and denatured nucleic acids: an experimental approach.
Völker J, Klump HH, Manning GS, Breslauer KJ. Völker J, et al. J Mol Biol. 2001 Jul 27;310(5):1011-25. doi: 10.1006/jmbi.2001.4841. J Mol Biol. 2001. PMID: 11501992
-
Prediction of melting profiles and local helix stability for sequenced DNA.
Gotoh O. Gotoh O. Adv Biophys. 1983;16:1-52. doi: 10.1016/0065-227x(83)90007-2. Adv Biophys. 1983. PMID: 6399811 Review.
Cited by
-
Single-molecule micromanipulation studies of methylated DNA.
Zaichuk T, Marko JF. Zaichuk T, et al. Biophys J. 2021 Jun 1;120(11):2148-2155. doi: 10.1016/j.bpj.2021.03.039. Epub 2021 Apr 8. Biophys J. 2021. PMID: 33838135 Free PMC article.
-
Prebiotic chemical origin of biomolecular complementarity.
Sajeev Y. Sajeev Y. Commun Chem. 2023 Nov 27;6(1):259. doi: 10.1038/s42004-023-01060-8. Commun Chem. 2023. PMID: 38012323 Free PMC article. Review.
-
Amangeldina A, Tan ZW, Berezovsky IN. Amangeldina A, et al. Curr Res Struct Biol. 2024 Feb 1;7:100129. doi: 10.1016/j.crstbi.2024.100129. eCollection 2024. Curr Res Struct Biol. 2024. PMID: 38327713 Free PMC article.
-
Protein-DNA recognition mechanisms and specificity.
Anashkina AA. Anashkina AA. Biophys Rev. 2023 Sep 7;15(5):1007-1014. doi: 10.1007/s12551-023-01137-7. eCollection 2023 Oct. Biophys Rev. 2023. PMID: 37974977 Free PMC article. Review.
-
Sandineni A, Lin B, MacKerell AD Jr, Cho BP. Sandineni A, et al. Chem Res Toxicol. 2013 Jun 17;26(6):937-51. doi: 10.1021/tx400116n. Epub 2013 Jun 4. Chem Res Toxicol. 2013. PMID: 23688347 Free PMC article.
References
-
- Marmur J., Doty P. Determination of the base composition of dioxyribonucleic acid from its thermal melting temperature. J. Mol. Biol. 1962;5:109–118. - PubMed
-
- Wartell R.M., Benight A.S. Thermal denaturation of DNA molecules. Phys. Rep. 1985;126:67–107.
-
- SantaLucia J., Jr, Hicks D. The thermodynamics of DNA structural motifs. Annu. Rev. Biophys. Biomol. Struct. 2004;33:415–440. - PubMed
-
- Williams M.C., Rouzina I. Force spectroscopy of single DNA and RNA molecules. Curr. Opin. Struct. Biol. 2002;12:330–336. - PubMed
-
- Vologodskii A.V., Amirikyan B.R., Lyubchenko Y.L., Frank-Kamenetskii M.D. Allowance for heterogeneous stacking in the DNA helix-coil transition theory. J. Biomol. Struct. Dyn. 1984;2:131–148. - PubMed
Publication types
MeSH terms
Substances
LinkOut - more resources
Full Text Sources
Other Literature Sources