Suppression of experimental autoimmune encephalomyelitis by extracellular adherence protein of Staphylococcus aureus - PubMed
- ️Sun Jan 01 2006
Suppression of experimental autoimmune encephalomyelitis by extracellular adherence protein of Staphylococcus aureus
Changping Xie et al. J Exp Med. 2006.
Abstract
Multiple sclerosis (MS) is a devastating inflammatory disorder of the central nervous system (CNS). A major hallmark of MS is the infiltration of T cells reactive against myelin components. T cell infiltration is mediated by the interaction of integrins of the beta1 and beta2 family expressed by lymphocytes with their endothelial counter-receptors, vascular cell adhesion molecule 1 and intercellular adhesion molecule (ICAM)-1, respectively. We have reported previously that extracellular adherence protein (Eap) of Staphylococcus aureus exerts antiinflammatory activities by interacting with ICAM-1 and blocking beta2-integrin-dependent neutrophil recruitment. Here, we report that Eap inhibits experimental autoimmune encephalomyelitis (EAE) in mice. In vitro, Eap reduced adhesion of peripheral blood T cells to immobilized ICAM-1 as well as their adhesion and transmigration of TNF-activated human endothelium under static and shear flow conditions. These inhibitory effects were corroborated in two mouse models of inflammation. In a delayed-type hypersensitivity model, both T cell infiltration and the corresponding tissue edema were significantly reduced by Eap. In addition, Eap administration prevented the development of EAE and markedly decreased infiltration of inflammatory cells into the CNS. Strikingly, intervention with Eap after the onset of EAE suppressed the disease. Collectively, our findings indicate that Eap represents an attractive treatment for autoimmune neuroinflammatory disorders such as MS.
Figures
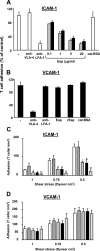
Eap inhibits T cell adhesion to ICAM-1. (A) The adhesion of T cells to immobilized ICAM-1 is shown in the absence (white bars) or presence of blocking mAb against VLA-4, mAb against LFA-1 (each at 20 μg/ml), increasing concentrations of purified (gray bars), or recombinant (black bars) Eap, or cationic BSA (20 μg/ml). (B) The adhesion of T cells to immobilized VCAM-1 is shown in the absence or presence of blocking mAb against VLA-4, mAb against LFA-1 (each at 20 μg/ml), purified Eap, recombinant Eap, or cationic BSA (each at 20 μg/ml). Cell adhesion is expressed relative to control (in the absence of competitor). Data are mean ± SD (n = 3) of a typical experiment; similar results were obtained in three separate experiments. (C and D) Adhesion of T cells to immobilized ICAM-1 (C) or VCAM-1 (D) under laminar shear flow conditions is shown in the absence (white bars) or presence of cationic BSA (diagonally striped bars), purified Eap (gray bars), recombinant Eap (black bars), or anti-LFA-1 mAb (dotted bars) (each at 20 μg/ml). Data are mean ± SEM, n = 3 separate experiments. *, P < 0.05. **, P < 0.01.
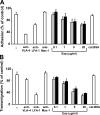
Eap inhibits T cell–endothelial cell interactions. (A) The adhesion of T cells to TNF-α prestimulated HUVEC (10 ng/ml, 16 h) is shown in the absence or presence of blocking mAb against VLA-4, blocking mAb against LFA-1, blocking mAb against Mac-1 (each at 20 μg/ml) (white bars), increasing concentrations of purified Eap (gray bars), recombinant Eap (black bars) or cationic BSA (20 μg/ml; white bars). (B) SDF-1α–stimulated transendothelial migration of T cells is shown in the absence (white bars) or presence of blocking mAb against VLA-4, blocking mAb against LFA-1, blocking mAb against Mac-1 (each at 20 μg/ml) (white bars), increasing concentrations of purified Eap (gray bars), recombinant Eap (black bars), or cationic BSA (20 μg/ml; white bars). Cell adhesion and cell transmigration is expressed relative to control (in the absence of competitor). Data are mean ± SD (n = 3) of a typical experiment; similar results were obtained in three separate experiments.
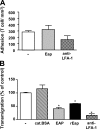
Effect of Eap on T cell–endothelial cell interactions under physiologic flow conditions. T cells were drawn across TNF-α–stimulated endothelial monolayers (25 ng/ml, 4 h) under laminar shear flow conditions (0.76 dynes/mm2). (A) The adhesion of T cells was studied without (white bar) or with purified Eap (gray bar) or anti–LFA-1 mAb (dotted bar) (each 20 μg/ml). (B) The transmigration of T cells was studied without (white bar) or with cationic BSA (diagonally striped bar), purified Eap (gray bar), recombinant Eap (black bar), or anti–LFA-1 mAb (dotted bar) (each 20 μg/ml). T cell adhesion (A) and transmigration relative to control (B) were determined as described in Materials and methods. Data are mean ± SEM, n = 3 separate experiments. *, P < 0.05.
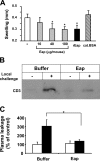
Inhibition of DTH response by Eap. (A) Mice were immunized with OXA (day 0) and at day 5 mice were challenged with local application of OXA on the ear. 2 h before local challenge, mice were treated without (white bar) or with increasing concentrations of purified Eap (gray bars), recombinant Eap (50 μg, black bar), or cationic BSA (100 μg, diagonally striped bar) administered intraperitoneally (n = 5 mice/group). Ear thickness was measured after 24 h. The difference in the ear thickness in mm is shown. Data are mean ± SD (n = 7). (B) The detection of infiltrated T cells is shown by detection of CD3 in Western blot. Ear tissues from DTH-induced mice that were treated with buffer or Eap were homogenized and the whole tissue lysates were subjected to Western blot to detect the amount of CD3. A typical Western blot for CD3 from the ears of buffer- or Eap-treated mice without (−) or with (+) local challenge using OXA is shown. (C) The vascular leakage (as assessed by influx of Evan's blue) of nonchallenged (white bars) or OXA challenged (black bars) ears is shown in mice pretreated with buffer (n = 5) or with Eap (n = 5). Ear tissue was removed, dried, and formamide was added to the dried ear tissue to extract Evan's blue; the optical density was registered at 590 nm, as a measure for the content of leaked Evan's blue. Plasma leakage is shown as percent of control (nonchallenged ear of buffer-treated mice) and data are mean ± SD (n = 5). *, P < 0.03.
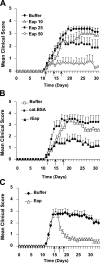
Inhibition of EAE by Eap. MOG-mediated EAE response in mice receiving different treatments. (A) Mice were treated with buffer (closed diamonds) or increasing concentrations of purified Eap (10 μg; open triangles; 25 μg; closed squares; 50 μg, open diamonds) that were administered on days 11, 14, and 17 (arrows) (n = 5–8 mice/group). (B) Mice were treated with buffer (open squares), recombinant Eap (50 μg, closed triangles), or cationic BSA (50 μg, closed squares) that were administered on days 11, 14, and 17 (arrows) (n = 5 mice/group). (C) Purified Eap (50 μg, open triangles) was administered on days 15, 17, and 19 (arrows) (i.e., after the onset of EAE; n = 5 mice/group). Data are represented as the mean clinical score and are mean ± SEM.
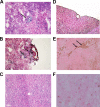
Eap inhibits the infiltration of the CNS by inflammatory cells during EAE. Histology (hematoxylin and eosin staining; A–D) and immunohistology (E–F) from brain sections of EAE mice. Typical photomicrographs indicating parenchymal (A) or perivascular (B) inflammatory cell infiltration in the CNS of buffer-treated mice during EAE. The arrows indicate the inflammatory cell infiltrates. No parenchymal or perivascular cell infiltration was noted in Eap-treated mice (C and D). Massive T cell infiltrates were noted in the brains of buffer-treated mice as indicated by the CD3 staining (E), whereas CD3-positive cells were hardly present in the brains of Eap-treated mice (F).
Similar articles
-
Wang H, von Rohrscheidt J, Roehrbein J, Peters T, Sindrilaru A, Kess D, Preissner KT, Scharffetter-Kochanek K. Wang H, et al. J Invest Dermatol. 2010 Mar;130(3):743-54. doi: 10.1038/jid.2009.310. Epub 2009 Oct 8. J Invest Dermatol. 2010. PMID: 19812597
-
Göbel K, Schuhmann MK, Pankratz S, Stegner D, Herrmann AM, Braun A, Breuer J, Bittner S, Ruck T, Wiendl H, Kleinschnitz C, Nieswandt B, Meuth SG. Göbel K, et al. Eur J Immunol. 2014 Aug;44(8):2295-305. doi: 10.1002/eji.201344107. Epub 2014 Jun 5. Eur J Immunol. 2014. PMID: 24811005
-
Kumar P, Kretzschmar B, Herold S, Nau R, Kreutzfeldt M, Schütze S, Bähr M, Hein K. Kumar P, et al. J Neuroinflammation. 2015 Feb 3;12:22. doi: 10.1186/s12974-015-0241-8. J Neuroinflammation. 2015. PMID: 25644616 Free PMC article.
-
Velázquez FE, Anastasiou M, Carrillo-Salinas FJ, Ngwenyama N, Salvador AM, Nevers T, Alcaide P. Velázquez FE, et al. Immunology. 2019 May;157(1):52-69. doi: 10.1111/imm.13047. Epub 2019 Feb 17. Immunology. 2019. PMID: 30690734 Free PMC article.
-
Chaudhary P, Marracci GH, Bourdette DN. Chaudhary P, et al. J Neuroimmunol. 2006 Jun;175(1-2):87-96. doi: 10.1016/j.jneuroim.2006.03.007. Epub 2006 Apr 27. J Neuroimmunol. 2006. PMID: 16644024
Cited by
-
Pathogenic Bacterial Proteins and their Anti-Inflammatory Effects in the Eukaryotic Host.
Sun J. Sun J. Antiinflamm Antiallergy Agents Med Chem. 2009 Sep 1;8(3):214-227. doi: 10.2174/187152309789151986. Antiinflamm Antiallergy Agents Med Chem. 2009. PMID: 20090866 Free PMC article.
-
Sá-Nunes A, Bafica A, Antonelli LR, Choi EY, Francischetti IM, Andersen JF, Shi GP, Chavakis T, Ribeiro JM, Kotsyfakis M. Sá-Nunes A, et al. J Immunol. 2009 Jun 15;182(12):7422-9. doi: 10.4049/jimmunol.0900075. J Immunol. 2009. PMID: 19494265 Free PMC article.
-
Novel Evasion Mechanisms of the Classical Complement Pathway.
Garcia BL, Zwarthoff SA, Rooijakkers SH, Geisbrecht BV. Garcia BL, et al. J Immunol. 2016 Sep 15;197(6):2051-60. doi: 10.4049/jimmunol.1600863. J Immunol. 2016. PMID: 27591336 Free PMC article. Review.
-
Sanchez JMS, Doty DJ, DePaula-Silva AB, Brown DG, Bell R, Klag KA, Truong A, Libbey JE, Round JL, Fujinami RS. Sanchez JMS, et al. J Neuroinflammation. 2020 Oct 6;17(1):291. doi: 10.1186/s12974-020-01959-2. J Neuroinflammation. 2020. PMID: 33023618 Free PMC article.
-
Sever-Chroneos Z, Krupa A, Davis J, Hasan M, Yang CH, Szeliga J, Herrmann M, Hussain M, Geisbrecht BV, Kobzik L, Chroneos ZC. Sever-Chroneos Z, et al. J Biol Chem. 2011 Feb 11;286(6):4854-70. doi: 10.1074/jbc.M110.125567. Epub 2010 Dec 1. J Biol Chem. 2011. PMID: 21123169 Free PMC article.
References
-
- ‘t Hart, B.A., and S. Amor. 2003. The use of animal models to investigate the pathogenesis of neuroinflammatory disorders of the central nervous system. Curr. Opin. Neurol. 16:375–383. - PubMed
-
- Springer, T.A. 1994. Traffic signals for lymphocyte recirculation and leukocyte emigration: the multistep paradigm. Cell. 76:301–314. - PubMed
-
- Yednock, T.A., C. Cannon, L.C. Fritz, F. Sanchez-Madrid, L. Steinman, and N. Karin. 1992. Prevention of experimental autoimmune encephalomyelitis by antibodies against α4 β1 integrin. Nature. 356:63–66. - PubMed
-
- Gordon, E.J., K.J. Myers, J.P. Dougherty, H. Rosen, and Y. Ron. 1995. Both anti-CD11a (LFA-1) and anti-CD11b (MAC-1) therapy delay the onset and diminish the severity of experimental autoimmune encephalomyelitis. J. Neuroimmunol. 62:153–160. - PubMed
Publication types
MeSH terms
Substances
Grants and funding
LinkOut - more resources
Full Text Sources
Other Literature Sources
Miscellaneous