Nas transgenic mouse line allows visualization of Notch pathway activity in vivo - PubMed
Nas transgenic mouse line allows visualization of Notch pathway activity in vivo
Céline Souilhol et al. Genesis. 2006 Jun.
Abstract
The Notch signaling pathway plays multiple and important roles in mammals. However, several aspects of its action, in particular, the precise mapping of its sites of activity, remain unclear. To address this issue, we generated a transgenic line carrying a construct consisting of a nls-lacZ reporter gene under the control of a minimal promoter and multiple RBP-Jkappa binding sites. Here we show that this transgenic line, which we termed NAS (for Notch Activity Sensor), displays an expression profile that is consistent with current knowledge on Notch activity sites in mice, even though it may not report on all these sites. Moreover, we observe that NAS transgene expression is abolished in a RBP-Jkappa-deficient background, indicating that it indeed requires Notch/RBP-Jkappa signaling pathway activity. Thus, the NAS transgenic line constitutes a valuable and versatile tool to gain further insights into the complex and various functions of the Notch signaling pathway.
Figures
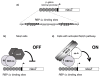
(a) Schematic representation of the TP1-nlacZ transgene. The nlacZ gene is under the control of the β-globin minimal promoter (black rectangle) preceded by six copies of a 50 bp long fragment (grey circle) from the promoter region of the Epstein-Barr virus TP1 gene. This fragment contains two RBP-Jκ binding sites (indicated in bold) and thus the TP1-nlacZ construct harbours 12 binding sites, (b, c) In absence of activated Notch (b), RBP-Jκ might recruit co-repressor complexes (co-R) to the TP1-nlacZ transgene which therefore should not be transcribed. When a cell receives signals through Notch receptor, RBP-Jκ/NICD, and co-factors (co-A) assemble into an activation complex leading to the transcription of the nlacZ gene.
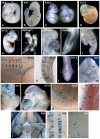
Whole mount X-gal staining of embryos of the following ages: E9.5 (a), E10 (b), E10.5 (c), and E11.5 (d). Arrows in (a,b) point to lacZ expressing cells in the cardiac region, (e) Internal view of a E12.5 NAS embryo cut in half, (f) RBP-JκΔ/Δ deficient embryos recovered 10 days after mating do not express the TP1-nlacZ transgene. Magnified views of regions indicated by boxes in (f) show the absence of IacZ expressing cells in cardiac (g) and tail (h) regions. Detail of the staining of the tail of E9.5 (i) and E11.5 (j, boxed region in (d)) NAS embryos. Arrows point to the band of β-gal positive cells in the PSM (k) Vibratome thick section of X-gal stained E11.5 tail showing a row of NAS expressing cells in the PSM (arrows) at the time when cleft starts to form (arrowhead). (l) Whole mount in situ hybridization of E10.5 tail showing that IacZ mRNA is detected essentially in the boundary forming region of the PSM (arrowhead). (m) Section in the trunk region (arrow in (b)) of E10 embryo showing TP1-nlacZ expression in the walls of dorsal aorta (da) and vitelline artery (va). ur: urogenital ridge. (n) Upper view of the heart region of E10.5 NAS embryo from which the head has been cut off. TP1-nlacZ expression is found in the distal portion of the outflow tract (oft) and in the branchial arch arteries (ba). nt: neural tube, (o–p) Enlarged views corresponding to boxed regions in (e) showing TP1-nlacZ expression in dorsal aorta (da) and caudal artery (ca) (p) and in head arteries (o) of E12.5 embryo. Arrow in (o) points to X-gal staining in the region of cortical hem (q) Detail of the X-gal staining of E17.5 NAS embryo. TP1-nlacZ expression is barely detectable in the dorsal aorta (ao) while it is clearly visible in the intercostal arteries (ica) branching directly from it. (r) Ventral view of dissected brain from a 4 weeks old NAS mouse showing the staining in cerebral arteries and in entorhinal cortex (arrow). Inset shows a magnified view of the branching of the right sylvian artery to the Willis polygon, (s–t) Details of pial arteries (s) and cerebral cortex microvessel (t) X-gal staining from 4 weeks old NAS mouse.
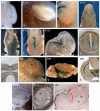
(a–c) Detail of the staining of the forelimb bud of E10 (a), E11.5 (b) and E12.5 (c) NAS embryos. (d) Frontal view of E11.5 NAS embryo showing labelling of the medio-caudal portion of cortical hemispheres. (e–f) Dorsal views showing X-gal staining in the hindbrain of E10.5 (e) and E11.5 (f) NAS embryos. Black arrows in (f) indicate the anterior and posterior limits of the medial bilateral stripe and the open arrow indicates the anterior limit of the lateral stripe. (g) Internal view of the head of E12.5 NAS embryo cut in half. Arrow points to a stripe of lacZ expressing cells in the neural tube, (h–i) Transverse sections of E11.5 NAS embryo in the trunk (h) and hindbrain (i) regions. Positions of sections along the antero-posterior embryonic axis are indicated by dotted lines in (f). (j) Md-sagittal view of cerebellum from 4 weeks old mouse showing TP1-nlacZ expression in Purkinje cell layer (arrow). Stripes of X-Gal labeled cells in the bulb (arrowheads) correspond to cerebral microvessels. (k) Whole mount X-gal staining of dissected brain from 4 weeks old NAS mouse. (I) Coronal section at the level indicated by a dotted line in (k) showing layer restricted expression of TP1-nlacZ in entorhinal cortex. Inset shows a magnified view of the TP1-nlacZ expressing region. (m) Internal view of the head of E17.5 embryo cut in half showing TP1-nlacZ expression in the labial surface of developing incisors. (n) Vibratome thick frontal section of X-gal stained E17.5 incisor bub showing NAS expression in stratum intermedium (si) layer, ame: amel oblast layer, odo: odontoblast layer, dp: dental papilla. (o) Microtome section of P6 developing molar. d: dentine.
Similar articles
-
Ikaros represses the transcriptional response to Notch signaling in T-cell development.
Kleinmann E, Geimer Le Lay AS, Sellars M, Kastner P, Chan S. Kleinmann E, et al. Mol Cell Biol. 2008 Dec;28(24):7465-75. doi: 10.1128/MCB.00715-08. Epub 2008 Oct 13. Mol Cell Biol. 2008. PMID: 18852286 Free PMC article.
-
Schouwey K, Aydin IT, Radtke F, Beermann F. Schouwey K, et al. Oncogene. 2011 Jan 20;30(3):313-22. doi: 10.1038/onc.2010.428. Epub 2010 Sep 20. Oncogene. 2011. PMID: 20856205
-
RBP-Jkappa-dependent notch signaling is dispensable for mouse early embryonic development.
Souilhol C, Cormier S, Tanigaki K, Babinet C, Cohen-Tannoudji M. Souilhol C, et al. Mol Cell Biol. 2006 Jul;26(13):4769-74. doi: 10.1128/MCB.00319-06. Mol Cell Biol. 2006. PMID: 16782866 Free PMC article.
-
Bertagna A, Toptygin D, Brand L, Barrick D. Bertagna A, et al. Biochem Soc Trans. 2008 Apr;36(Pt 2):157-66. doi: 10.1042/BST0360157. Biochem Soc Trans. 2008. PMID: 18363556 Review.
-
Selective repression of Notch pathway target gene transcription.
Cave JW. Cave JW. Dev Biol. 2011 Dec 1;360(1):123-31. doi: 10.1016/j.ydbio.2011.09.018. Epub 2011 Sep 22. Dev Biol. 2011. PMID: 21963536 Review.
Cited by
-
Vilas-Boas F, Fior R, Swedlow JR, Storey KG, Henrique D. Vilas-Boas F, et al. BMC Biol. 2011 Aug 31;9:58. doi: 10.1186/1741-7007-9-58. BMC Biol. 2011. PMID: 21880129 Free PMC article.
-
Monet-Leprêtre M, Bardot B, Lemaire B, Domenga V, Godin O, Dichgans M, Tournier-Lasserve E, Cohen-Tannoudji M, Chabriat H, Joutel A. Monet-Leprêtre M, et al. Brain. 2009 Jun;132(Pt 6):1601-12. doi: 10.1093/brain/awp049. Epub 2009 Mar 17. Brain. 2009. PMID: 19293235 Free PMC article.
-
Notch Signaling and the Skeleton.
Zanotti S, Canalis E. Zanotti S, et al. Endocr Rev. 2016 Jun;37(3):223-53. doi: 10.1210/er.2016-1002. Epub 2016 Apr 13. Endocr Rev. 2016. PMID: 27074349 Free PMC article. Review.
-
Payne S, Gunadasa-Rohling M, Neal A, Redpath AN, Patel J, Chouliaras KM, Ratnayaka I, Smart N, De Val S. Payne S, et al. Nat Commun. 2019 Jul 22;10(1):3276. doi: 10.1038/s41467-019-10710-2. Nat Commun. 2019. PMID: 31332177 Free PMC article.
-
Genetic visualization of notch signaling in mammalian neurogenesis.
Imayoshi I, Shimojo H, Sakamoto M, Ohtsuka T, Kageyama R. Imayoshi I, et al. Cell Mol Life Sci. 2013 Jun;70(12):2045-57. doi: 10.1007/s00018-012-1151-x. Epub 2012 Sep 13. Cell Mol Life Sci. 2013. PMID: 22971775 Free PMC article. Review.
References
-
- Artavanis-Tsakonas S, Matsuno K, Fortini ME. Notch signaling. Science. 1995;268:225–232. - PubMed
-
- Artavanis-Tsakonas S, Rand MD, Lake RJ. Notch signaling: cell fate control and signal integration in development. Science. 1999;284:770–776. - PubMed
-
- Aulehla A, Herrmann BG. Segmentation in vertebrates: clock and gradient finally joined. Genes Dev. 2004;18:2060–2067. - PubMed
-
- Barrantes IB, Elia AJ, Wunsch K, Hrabe de Angelis MH, Mak TW, Rossant J, Conlon RA, Gossler A, de la Pampa JL. Interaction between Notch signalling and Lunatic fringe during somite boundary formation in the mouse. Curr Biol. 1999;9:470–480. - PubMed
Publication types
MeSH terms
Substances
LinkOut - more resources
Full Text Sources
Other Literature Sources
Molecular Biology Databases