EphB2 and EphB4 receptors forward signaling promotes SDF-1-induced endothelial cell chemotaxis and branching remodeling - PubMed
- ️Sun Jan 01 2006
EphB2 and EphB4 receptors forward signaling promotes SDF-1-induced endothelial cell chemotaxis and branching remodeling
Ombretta Salvucci et al. Blood. 2006.
Abstract
The complex molecular mechanisms that drive endothelial cell movement and the formation of new vessels are poorly understood and require further investigation. Eph receptor tyrosine kinases and their membrane-anchored ephrin ligands regulate cell movements mostly by cell-cell contact, whereas the G-protein-coupled receptor CXCR4 and its unique SDF-1 chemokine ligand regulate cell movement mostly through soluble gradients. By using biochemical and functional approaches, we investigated how ephrinB and SDF-1 orchestrate endothelial cell movement and morphogenesis into capillary-like structures. We describe how endogenous EphB2 and EphB4 signaling are required for the formation of extracellular matrix-dependent capillary-like structures in primary human endothelial cells. We further demonstrate that EphB2 and EphB4 activation enhance SDF-1-induced signaling and chemotaxis that are also required for extracellular matrix-dependent endothelial cell clustering. These results support a model in which SDF-1 gradients first promote endothelial cell clustering and then EphB2 and EphB4 critically contribute to subsequent cell movement and alignment into cord-like structures. This study reveals a requirement for endogenous Eph signaling in endothelial cell morphogenic processes, uncovers a novel link between EphB forward signaling and SDF-1-induced signaling, and demonstrates a mechanism for cooperative regulation of endothelial cell movement.
Figures
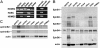
Expression of EphB receptors and ephrin B ligands in primary human endothelial cells from the umbilical vein, aorta, and dermal microvasculature. (A) EphB1, EphB2, EphB3, EphB4, and EphB6 receptor and ephrin B1, ephrin B2, and ephrin B3 ligand mRNA detected by semiquantitative RT-PCR with specific primer pairs in HUVECs, HAECs, and HDMECs. Representative results from 3 experiments. (B) EphB1, EphB2, EphB3, EphB4, and EphB6 were detected by Western blotting with specific antibodies in cell lysates from murine NIH3T3 cells, human brain tissue (brain), HUVEC, human SK-N-MC neuroblastoma cell line (neuro), human A-204 rhabdomyosarcoma cell line (rhabd), and human SK-NEP-1 Wilms tumor cell line (wilms), HAEC, and HDMEC. Results shown, which are representative of 4 independent immunoblotting experiments, reflect reprobing of a single membrane after stripping; sample loading was evaluated by reprobing for actin. (C) Immunoblotting analysis of ephrin B2 and ephrin B3 expression in HUVECs, HAECs, HDMECs, and other samples—murine NIH3T3 cells, human brain tissue (brain), human SK-N-MC neuroblastoma cell line (neuro), human A-204 rhabdomyosarcoma cell line (rhabd), and human SK-NEP-1 Wilms tumor cell line (wilms). A single membrane was used for immune detection; sample loading was evaluated by probing with antiactin antibodies. Results are representative of 3 immunoblotting experiments.
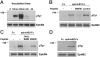
Detection of EphB2 and EphB4 phosphorylation in primary human endothelial cells. (A) Kinetics of EphB2 phosphorylation in HUVECs stimulated with ephrin B1-Fc. HUVECs were incubated in medium only or were treated with ephrin B1-Fc (1 μg/mL) for 10 minutes, 30 minutes, 2 hours, and 4 hours. Cell lysates (150 μg) were immunoprecipitated with goat IgG antibodies to EphB2, and the precipitates were immunoblotted with anti–phosphotyrosine mouse monoclonal antibodies (4G10). The membrane was reblotted with goat IgG antibodies to EphB2 (results are representative of 3 experiments). (B) Effect of the SNEW peptide (100 μM) on EphB2 phosphorylation in HUVECs stimulated for 10 minutes with ephrin B1-Fc (1 μg/mL) or with a control Fc-fusion protein (Fc, leptin receptor-Fc chimera, 1 μg/mL). As a control peptide (control), we used a scrambled form of SNEW (SCR-EPQ, 100 μM). EphB2 was immunoprecipitated, immunoblotted with anti–phosphotyrosine mouse monoclonal antibodies, and reblotted with goat IgG antibodies to EphB2 antibodies. (C) EphB4 phosphorylation was induced in HUVECs stimulated with ephrin B2-Fc (1 μg/mL). HUVECs were incubated for 30 minutes with a control Fc-fusion protein (Fc, leptin receptor-Fc chimera, 1 μg/mL) or with ephrin B2-Fc (1 μg/mL) in medium alone or in medium supplemented with the peptide TNYL-RAW (100 μM) or the peptide SNEW (100 μM). EphB4 was immunoprecipitated, immunoblotted with anti–phosphotyrosine mouse monoclonal antibodies, and reblotted with goat IgG antibodies to EphB4 antibodies. (D) EphB2 phosphorylation was induced in HUVECs after stimulation for 30 minutes with ephrin B2-Fc (1 μg/mL) but not with a control Fc-fusion protein (Fc, leptin receptor-Fc chimera, 1 μg/mL). Phosphorylated EphB2 was visualized after immunoprecipitation and immunoblotting with anti–phosphotyrosine mouse monoclonal antibodies; total EphB2 was visualized after reprobing with antibodies to EphB2.
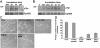
Analysis of EphB2 and EphB4 phosphorylation and function during endothelial cell cord formation on extracellular matrix. (A-B) HUVECs were incubated on Matrigel-coated wells for the indicated times. After incubation, cells were washed in PBS at 4°C and removed by trypsin digestion at 4°C, and cell lysates were immunoprecipitated with goat IgG antibodies to EphB2 (A) or EphB4 (B). Immunoprecipitates were immunoblotted with anti–phosphotyrosine mouse monoclonal antibody 4G10, and the membranes were reblotted with the immunoprecipitating antibodies to EphB2 or EphB4. Results derived from independent experiments, each representative of 3 experiments. (C) Effects of the peptides CTCE-9908, SNEW, and TNYL-RAW on Matrigel-dependent cord formation. HUVECs were incubated for 18 hours onto Matrigel-coated wells in medium alone (none), with control peptide SCR-WTL (100 μM), CTCE-9908 peptide (50 μg/mL), SNEW peptide (100 μM), or TNYL-RAW peptide (100 μM). Representative images from phase-contrast microscopy reflecting various degrees of cord formation after 18 hours of incubation (original magnification, ×5). Representative results from 5 experiments. (D) Quantitative analysis of HUVEC cord formation on Matrigel-coated wells under the same conditions listed in panel C and measured as a function of the number of vascular joints per visual field (magnification, ×10). Results reflect the mean ± SD of 3 independent experiments; in each experiment, 4 nonoverlapping fields were counted.
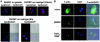
Surface EphB2 and EphB4 expression and actin polymerization during cord formation. (A) HUVECs were plated onto gelatin-coated or Matrigel-coated glass chamber slides and incubated at 37°C. After 45 minutes and 6 hours of incubation, cells were fixed with 1% formaldehyde, stained for EphB2 or EphB4 with specific goat IgG antibodies followed by Texas Red donkey anti–goat IgG and DAPI, and examined with a confocal system. Images were from phase-contrast and epifluorescence microscopy showing representative HUVECs cultured on gelatin (18 hours) or Matrigel (45 minutes and 6 hours) (original magnification, ×10 ×25). (B) HUVECs were plated onto Matrigel-coated glass chamber slides and were incubated at 37°C in medium alone, medium with the SNEW peptide (100 μM), or medium with the TNYL-RAW peptide (100 μM) for 6 hours and then stained with phalloidin-FITC and DAPI. Images from confocal epifluorescence microscopy show representative F-actin detection in HUVECs (original magnification, ×25).
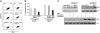
Akt phosphorylation in HUVECs stimulated with SDF-1, ephrin B1-Fc, and ephrin B2-Fc. (A) Flow cytometric analysis of intracellular Akt in HUVECs incubated for 15 minutes in medium alone, SDF-1α (500 ng/mL), ephrin B1-Fc (1 μg/mL), ephrin B2-Fc (1 μg/mL), ephrin B1-Fc (1 μg/mL) plus SDF-1α (500 ng/mL), or ephrin B2-Fc (1 μg/mL) plus SDF-1α (500 ng/mL). After permeabilization, Akt was detected by staining with a rabbit monoclonal antibody to phospho-Akt (Ser 473) followed by FITC-labeled goat anti–rabbit IgG antibodies. Background staining was set with FITC-labeled goat anti–rabbit IgG. FSC indicates forward scatter. Results are representative of 5 experiments. (B) Flow cytometric analysis of intracellular Akt. HUVECs were incubated for 15 minutes with ephrin B1-Fc (1 μg/mL) plus SDF-1α (500 ng/mL) alone, with the SNEW peptide (100 μM) or with a control peptide (SCR-EPQ, a scrambled form of SNEW [100 μM]), with ephrin B2-Fc (1 μg/mL) plus SDF-1α (500 ng/mL) alone, with the TNYL-RAW peptide (100 μM), or with a control peptide (SCR-WTL, a scrambled form of TNYL-RAW, 100 μM). (C, top left) After 3-hour starvation, HUVECs were treated with SDF-1α (500 ng/mL), ephrin B1-Fc (1 μg/mL), or ephrin B1-Fc (1 μg/mL) plus SDF-1α (500 ng/mL) for 15 minutes. (C, top right) After 3-hour starvation, HUVECs were treated with SDF-1 (500 ng/mL), ephrin B2-Fc (1 μg/mL), or ephrin B2-Fc (1 μg/mL) plus SDF-1α (500 ng/mL) for 15 minutes. (C, bottom) After overnight starvation, HUVECs were treated for 15 minutes with SDF-1 (100 ng/mL), ephrin B1-Fc (1 μg/mL), ephrin B2-Fc (1 μg/mL), ephrin B1-Fc (1 μg/mL) plus ephrin B2-Fc (1 μg/mL), ephrin B1-Fc (1 μg/mL) plus SDF-1α (100 ng/mL), ephrin B2-Fc (1 μg/mL) plus SDF-1α (100 ng/mL), or with SDF-1 (100 ng/mL) plus ephrin B1-Fc (1 μg/mL) plus ephrin B2-Fc (1 μg/mL). Cell lysates were immunoblotted with a rabbit monoclonal antibody to phospho-Akt (Ser473) and were reblotted with rabbit antibodies against total Akt.
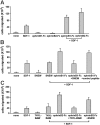
Stimulation with ephrin B1-Fc or ephrin B2-Fc enhances SDF-1-induced HUVEC chemotaxis. (A) HUVECs (0.5 × 106) preincubated (10 minutes, 37°C) in medium only, with ephrin B1-Fc (1 μg/mL), or with ephrin B2-Fc (1 μg/mL) were placed on the top chamber of the transwell. SDF-1α (50 ng/mL) or chemotaxis medium only was placed in the bottom chamber. (B) HUVECs (0.5 × 106) were first incubated (20 minutes) in medium only, with the peptide SNEW (100 μM), or with a control scrambled form of SNEW (SCR-EPQ, 100 μM). Cells were subsequently incubated (10 minutes, 37°C) with medium only or with ephrin B1-Fc (1 μg/mL). Cells and additives were placed on the top chamber of the transwell. SDF-1α (50 ng/mL), or chemotaxis medium only was placed in the bottom chamber, and the transwells were incubated for 18 hours at 37°C. (C) HUVECs (0.5 × 106) were first incubated (20 minutes, 37°C) in medium only, with the peptide TNYL-RAW (100 μM), or with the peptide SNEW (100 μM); cells were subsequently incubated (10 minutes, 37°C) with medium only or with ephrin B2-Fc (1 μg/mL). Cells and additives were placed on the top chamber of the transwell. SDF-1α (50 ng/mL) or chemotaxis medium only was placed in the bottom chamber. All transwell plates (A-C) were incubated 18 hours at 37°C. Cells accumulated into the lower chamber were counted. Results reflect the mean ± SD number of migrated HUVECs under the different conditions tested (3-5 separate experiments, each performed in triplicate).
Similar articles
-
Kim I, Ryu YS, Kwak HJ, Ahn SY, Oh JL, Yancopoulos GD, Gale NW, Koh GY. Kim I, et al. FASEB J. 2002 Jul;16(9):1126-8. doi: 10.1096/fj.01-0805fje. Epub 2002 May 21. FASEB J. 2002. PMID: 12039842
-
Li M, Yu J, Li Y, Li D, Yan D, Qu Z, Ruan Q. Li M, et al. Exp Mol Pathol. 2010 Apr;88(2):250-5. doi: 10.1016/j.yexmp.2009.12.001. Epub 2009 Dec 16. Exp Mol Pathol. 2010. PMID: 20025867
-
Interplay between EphB4 on tumor cells and vascular ephrin-B2 regulates tumor growth.
Noren NK, Lu M, Freeman AL, Koolpe M, Pasquale EB. Noren NK, et al. Proc Natl Acad Sci U S A. 2004 Apr 13;101(15):5583-8. doi: 10.1073/pnas.0401381101. Epub 2004 Apr 5. Proc Natl Acad Sci U S A. 2004. PMID: 15067119 Free PMC article.
-
Cy5.5-Anti-ephrin receptor B4 (EphB4) humanized monoclonal antibody hAb47.
Leung K. Leung K. 2013 Mar 18 [updated 2013 May 9]. In: Molecular Imaging and Contrast Agent Database (MICAD) [Internet]. Bethesda (MD): National Center for Biotechnology Information (US); 2004–2013. 2013 Mar 18 [updated 2013 May 9]. In: Molecular Imaging and Contrast Agent Database (MICAD) [Internet]. Bethesda (MD): National Center for Biotechnology Information (US); 2004–2013. PMID: 23678520 Free Books & Documents. Review.
-
CXCR4-SDF-1 signalling, locomotion, chemotaxis and adhesion.
Kucia M, Jankowski K, Reca R, Wysoczynski M, Bandura L, Allendorf DJ, Zhang J, Ratajczak J, Ratajczak MZ. Kucia M, et al. J Mol Histol. 2004 Mar;35(3):233-45. doi: 10.1023/b:hijo.0000032355.66152.b8. J Mol Histol. 2004. PMID: 15339043 Review.
Cited by
-
Targeting the Eph System with Peptides and Peptide Conjugates.
Riedl SJ, Pasquale EB. Riedl SJ, et al. Curr Drug Targets. 2015;16(10):1031-47. doi: 10.2174/1389450116666150727115934. Curr Drug Targets. 2015. PMID: 26212263 Free PMC article. Review.
-
Assembly and patterning of the vascular network of the vertebrate hindbrain.
Fujita M, Cha YR, Pham VN, Sakurai A, Roman BL, Gutkind JS, Weinstein BM. Fujita M, et al. Development. 2011 May;138(9):1705-15. doi: 10.1242/dev.058776. Epub 2011 Mar 23. Development. 2011. PMID: 21429985 Free PMC article.
-
Pennisi A, Ling W, Li X, Khan S, Shaughnessy JD Jr, Barlogie B, Yaccoby S. Pennisi A, et al. Blood. 2009 Aug 27;114(9):1803-12. doi: 10.1182/blood-2009-01-201954. Epub 2009 Jul 13. Blood. 2009. PMID: 19597185 Free PMC article.
-
Recent advances of the Ephrin and Eph family in cardiovascular development and pathologies.
Zhu Y, Su SA, Shen J, Ma H, Le J, Xie Y, Xiang M. Zhu Y, et al. iScience. 2024 Jul 19;27(8):110556. doi: 10.1016/j.isci.2024.110556. eCollection 2024 Aug 16. iScience. 2024. PMID: 39188984 Free PMC article. Review.
-
Shi W, Ye B, Rame M, Wang Y, Cioca D, Reibel S, Peng J, Qi S, Vitale N, Luo H, Wu J. Shi W, et al. J Biol Chem. 2020 May 29;295(22):7653-7668. doi: 10.1074/jbc.RA120.013251. Epub 2020 Apr 22. J Biol Chem. 2020. PMID: 32321761 Free PMC article.
References
-
- Pasquale EB. Eph receptor signalling casts a wide net on cell behaviour. Nat Rev Mol Cell Biol. 2005;6: 462-475. - PubMed
-
- Himanen JP, Chumley MJ, Lackmann M, et al. Repelling class discrimination: ephrin-A5 binds to and activates EphB2 receptor signaling. Nat Neurosci. 2004;7: 501-509. - PubMed
-
- Pasquale EB. Eph-ephrin promiscuity is now crystal clear. Nat Neurosci. 2004;7: 417-418. - PubMed
-
- Chrencik JE, Brooun A, Recht MI, et al. Structure and thermodynamic characterization of the EphB4/Ephrin-B2 antagonist peptide complex reveals the determinants for receptor specificity. Structure. 2006;14: 321-330. - PubMed
-
- Takemoto M, Fukuda T, Sonoda R, Murakami F, Tanaka H, Yamamoto N. Ephrin-B3-EphA4 interactions regulate the growth of specific thalamocortical axon populations in vitro. Eur J Neurosci. 2002;16: 1168-1172. - PubMed
Publication types
MeSH terms
Substances
LinkOut - more resources
Full Text Sources
Other Literature Sources
Research Materials
Miscellaneous