Hydride transfer catalysed by Escherichia coli and Bacillus subtilis dihydrofolate reductase: coupled motions and distal mutations - PubMed
- ️Sun Jan 01 2006
Comparative Study
Hydride transfer catalysed by Escherichia coli and Bacillus subtilis dihydrofolate reductase: coupled motions and distal mutations
Sharon Hammes-Schiffer et al. Philos Trans R Soc Lond B Biol Sci. 2006.
Abstract
This paper reviews the results from hybrid quantum/classical molecular dynamics simulations of the hydride transfer reaction catalysed by wild-type (WT) and mutant Escherichia coli and WT Bacillus subtilis dihydrofolate reductase (DHFR). Nuclear quantum effects such as zero point energy and hydrogen tunnelling are significant in these reactions and substantially decrease the free energy barrier. The donor-acceptor distance decreases to ca 2.7 A at transition-state configurations to enable the hydride transfer. A network of coupled motions representing conformational changes along the collective reaction coordinate facilitates the hydride transfer reaction by decreasing the donor-acceptor distance and providing a favourable geometric and electrostatic environment. Recent single-molecule experiments confirm that at least some of these thermally averaged equilibrium conformational changes occur on the millisecond time-scale of the hydride transfer. Distal mutations can lead to non-local structural changes and significantly impact the probability of sampling configurations conducive to the hydride transfer, thereby altering the free-energy barrier and the rate of hydride transfer. E. coli and B. subtilis DHFR enzymes, which have similar tertiary structures and hydride transfer rates with 44% sequence identity, exhibit both similarities and differences in the equilibrium motions and conformational changes correlated to hydride transfer, suggesting a balance of conservation and flexibility across species.
Figures
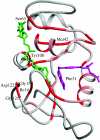
Three-dimensional structure of E. coli DHFR. The residues conserved across numerous species from E. coli to human are indicated by a gradient colour scheme (grey to red, where red is the most conserved). NADPH and DHF are in green and magenta, respectively. Reproduced with permission from Agarwal et al. (2002b).

The hydride transfer reaction from the NADPH cofactor to the H3F+ substrate.
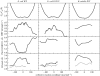
Selected thermally averaged geometrical properties along the collective reaction coordinate for WT E. coli (left), G121V mutant E. coli (middle) and WT B. subtilis (right) DHFR. From top to bottom, the properties plotted are: the donor–acceptor distance; the angle between the acceptor and methylene amino linkage in H2F; the distance between Cζ of Phe31 and C11 of H2F; the hydrogen-bonding distance between N of Asp122 and O of Gly15 (black) and between O of Ile14 and carboxamide N of NADPH (grey). The relevant residues are labelled in figure 1. The WT E. coli data were obtained from Agarwal et al. (2002b), the G121V mutant E. coli data were obtained from Watney et al. (2003), and the B. subtilis data were obtained from Watney & Hammes-Schiffer (2005). An improved numerical method for calculating the thermally averaged properties was used for the analysis of the B. subtilis data. The B. subtilis results obtained with the numerical method used to generate the E. coli results are provided in Watney (2005), and the qualitative trends are the same.
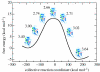
Free-energy profile of the DHFR-catalysed hydride transfer reaction as a function of a collective reaction coordinate that includes motions of the enzyme, substrate and cofactor. The magnitude of the free-energy barrier is determined by the relative probabilities of sampling the transition state and the reactant configurations. The thermally averaged equilibrium structures, as well as the average donor–acceptor distances in angstroms, are provided for selected values of the reaction coordinate. Note that the donor–acceptor distance decreases as the reaction evolves from the reactant to the transition state. The conformational changes along the collective reaction coordinate are attained by equilibrium thermal motions occurring within the confines of the protein fold and reflect a network of coupled motions extending throughout the protein. This network facilitates the hydride transfer reaction by bringing the donor and acceptor closer together, orienting the substrate and cofactor properly and providing a favourable electrostatic environment.
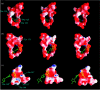
Electrostatic potential mapped to the molecular surface of the (a) and (b) βF-βG loop and (c) βG-βH loop. The potentials are given for the reactant state (left), transition state (middle) and product state (right). Blue indicates positive potential and red indicates negative potential. The substrate and cofactor are yellow and green, respectively. This figure was created using GRASP (Nicholls et al. 1991). Reproduced with permission from Wong et al. (2004).
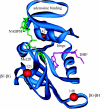
Three-dimensional structure of WT E. coli DHFR with the NADPH cofactor shown in green and the substrate shown in magenta. The important loop regions are identified and the residues involved in the mutant DHFR enzymes studied are labelled with red spheres. Reproduced with permission from Hammes-Schiffer (2006).
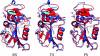
Thermally averaged structures for E. coli (red) and B. subtilis (blue) DHFR at the reactant state (RS), transition state (TS) and product state (PS). Reproduced with permission from Watney & Hammes-Schiffer (2005).
Similar articles
-
Comparison of coupled motions in Escherichia coli and Bacillus subtilis dihydrofolate reductase.
Watney JB, Hammes-Schiffer S. Watney JB, et al. J Phys Chem B. 2006 May 25;110(20):10130-8. doi: 10.1021/jp0605956. J Phys Chem B. 2006. PMID: 16706474
-
Wong KF, Selzer T, Benkovic SJ, Hammes-Schiffer S. Wong KF, et al. Proc Natl Acad Sci U S A. 2005 May 10;102(19):6807-12. doi: 10.1073/pnas.0408343102. Epub 2005 Apr 5. Proc Natl Acad Sci U S A. 2005. PMID: 15811945 Free PMC article.
-
Freezing a single distal motion in dihydrofolate reductase.
Sergi A, Watney JB, Wong KF, Hammes-Schiffer S. Sergi A, et al. J Phys Chem B. 2006 Feb 9;110(5):2435-41. doi: 10.1021/jp056939u. J Phys Chem B. 2006. PMID: 16471835
-
Catalytic efficiency of enzymes: a theoretical analysis.
Hammes-Schiffer S. Hammes-Schiffer S. Biochemistry. 2013 Mar 26;52(12):2012-20. doi: 10.1021/bi301515j. Epub 2012 Dec 20. Biochemistry. 2013. PMID: 23240765 Free PMC article. Review.
Cited by
-
Structural and mechanistic insights into Streptococcus pneumoniae NADPH oxidase.
Dubach VRA, San Segundo-Acosta P, Murphy BJ. Dubach VRA, et al. Nat Struct Mol Biol. 2024 Nov;31(11):1769-1777. doi: 10.1038/s41594-024-01348-w. Epub 2024 Jul 22. Nat Struct Mol Biol. 2024. PMID: 39039317 Free PMC article.
-
Second-Shell Residues Contribute to Catalysis by Predominately Preorganizing the Apo State in PafA.
Deng J, Cui Q. Deng J, et al. J Am Chem Soc. 2023 May 24;145(20):11333-11347. doi: 10.1021/jacs.3c02423. Epub 2023 May 12. J Am Chem Soc. 2023. PMID: 37172218 Free PMC article.
-
Structural insights into the octamerization of glycerol dehydrogenase.
Park T, Kang JY, Jin M, Yang J, Kim H, Noh C, Jung CH, Eom SH. Park T, et al. PLoS One. 2024 Mar 14;19(3):e0300541. doi: 10.1371/journal.pone.0300541. eCollection 2024. PLoS One. 2024. PMID: 38483875 Free PMC article.
-
Linking protein motion to enzyme catalysis.
Singh P, Abeysinghe T, Kohen A. Singh P, et al. Molecules. 2015 Jan 13;20(1):1192-209. doi: 10.3390/molecules20011192. Molecules. 2015. PMID: 25591120 Free PMC article. Review.
-
H-transfers in Photosystem II: what can we learn from recent lessons in the enzyme community?
Hay S, Scrutton NS. Hay S, et al. Photosynth Res. 2008 Oct-Dec;98(1-3):169-77. doi: 10.1007/s11120-008-9326-x. Epub 2008 Sep 3. Photosynth Res. 2008. PMID: 18766465 Review.
References
-
- Agarwal P.K, Billeter S.R, Hammes-Schiffer S. Nuclear quantum effects and enzyme dynamics in dihydrofolate reductase catalysis. J. Phys. Chem. B. 2002a;106:3283–3293. doi:10.1021/jp020190v - DOI
-
- Agarwal P.K, Billeter S.R, Rajagopalan P.T.R, Benkovic S.J, Hammes-Schiffer S. Network of coupled promoting motions in enzyme catalysis. Proc. Natl Acad. Sci. USA. 2002b;99:2794–2799. doi:10.1073/pnas.052005999 - DOI - PMC - PubMed
-
- Anderson J.B. Statistical theories of chemical reactions. Distributions in the transition region. J. Chem. Phys. 1973;58:4684–4692. doi:10.1063/1.1679032 - DOI
-
- Antikainen N.M, Smiley R.D, Benkovic S.J, Hammes G.G. Conformation coupled enzyme catalysis: single molecule and transient kinetics investigation of dihydrofolate reductase. Biochemistry. 2005;44:16 835–16 843. - PubMed
-
- Benkovic S.J, Hammes-Schiffer S. A perspective on enzyme catalysis. Science. 2003;301:1196–1202. doi:10.1126/science.1085515 - DOI - PubMed
Publication types
MeSH terms
Substances
LinkOut - more resources
Full Text Sources
Other Literature Sources