A 5' RNA element promotes dengue virus RNA synthesis on a circular genome - PubMed
- ️Sun Jan 01 2006
A 5' RNA element promotes dengue virus RNA synthesis on a circular genome
Claudia V Filomatori et al. Genes Dev. 2006.
Abstract
The mechanisms of RNA replication of plus-strand RNA viruses are still unclear. Here, we identified the first promoter element for RNA synthesis described in a flavivirus. Using dengue virus as a model, we found that the viral RdRp discriminates the viral RNA by specific recognition of a 5' element named SLA. We demonstrated that RNA-RNA interactions between 5' and 3' end sequences of the viral genome enhance dengue virus RNA synthesis only in the presence of an intact SLA. We propose a novel mechanism for minus-strand RNA synthesis in which the viral polymerase binds SLA at the 5' end of the genome and reaches the site of initiation at the 3' end via long-range RNA-RNA interactions. These findings provide an explanation for the strict requirement of dengue virus genome cyclization during viral replication.
Figures
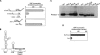
RNA discrimination by NS5pol is mediated by an RNA structure present at the 5′ end of the DV genome. (A) In vitro RdRp activity of the viral NS5pol using cellular and viral RNA templates. Schematic representations of the RNAs used are indicated on the left: (5′–3′DV) Subgenomic RNA encoding DV structural proteins flanked by the viral 5′ and 3′ UTRs, (5′–3′βGlo) mRNA encoding βglobin, (5′HCV) 220′ nt RNA of HCV 5′ UTR, (3′DV) 454′ nt DV 3′ UTR, and (5′DV) 5′-end 160 nt of DV genome. RNA polymerase activity was carried out as described in Materials and Methods. (B) Schematic representation of the predicted secondary structure of an RNA encompassing the first 100 nt of the DV genome obtained by Mfold algorithm. Stem–loop A (SLA), stem–loop B (SLB), and the complementary sequence (5′CS) within the viral coding sequence are indicated. (C) Requirement of SLA for NS5pol activity in vitro. Analysis of radiolabeled RNA products in denaturing 5% polyacrylamide gels synthesized by NS5pol. 5′DV RNAs with mutations within SLA, SLB, and CS (MSLA, MSLB, and MCS) or deletions of the complete structures (ΔSLA, ΔSLB, ΔCS, and ΔSLB-CS) were used at 300 nM as templates. (D) In vitro RNA synthesis by DV NS5pol using unrelated RNA templates encoding firefly luciferase preceded or not by DV SLA (SLA-Luc and Luc, respectively). RNA polymerase activity was expressed as described in A.
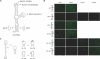
Stem–loop A is required for DV replication in BHK cells. (A) Schematic representation of specific mutations within SLA are indicated with arrows, and the nucleotide changes are shown for each mutant MS10, MS11, ML338, ML340, and M342. (B) Expression of DV proteins in cells transfected with wild-type and mutated viral RNAs was monitored by IFA. (C) Sequence of revertant viruses obtained after transfecting BHK cells with full-length DV RNA carrying the mutations ML338 and ML340 at the top loop of SLA. (Bold) Nucleotide substitutions of ML338 and ML340 RNAs, (gray circles) sequences of revertant viruses, Rev 338 and Rev 340.
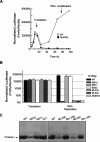
Stem–loop A is required for DV replicon RNA amplification in BHK cells. (A) Time course of luciferase activity showing the replication of the DV replicon in BHK cells. The times used to assess translation of input RNA or RNA amplification are shown by arrows. (B) Translation and RNA replication of wild-type and mutant DV replicons carrying mutations within SLA described in Fig. 2A (MS10, MS11, M342, ML338, and ML340) and the replication-incompetent MutNS5 were evaluated in BHK cells. Normalized luciferase levels are shown in logarithmic scale for each replicon RNA (DVRep) as indicated on the right. (C) In vitro NS5pol activity using 5′DVRNA templates corresponding to the first 160 nt of the DV genome with specific mutations around SLA. 5′DV RNAs with mutations within the stem of SLA (MS10 and MS11), the top loop of SLA (ML338 and ML340), and the 5′DV RNAs carrying the sequences from the revertant viruses (Rev 338 and Rev 340) as indicated on the top were used at 300 nM concentration as templates for the in vitro activity of viral NS5pol.
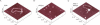
Visualization of RNA-NS5pol interaction by AFM. Twenty microliters of samples containing RNA (1ng/μL) and/or NS5pol(0.4ng/μL) was deposited on freshly cleaved mica for imaging analysis. (A) A representative RNA molecule in a circular conformation is observed (Alvarez et al. 2005b). This 2.3′kb RNA contains the 5′ and 3′ end sequences of DV flanking the sequence of the luciferase, which is annealed to a 1633′nt antisense RNA. In this RNA, the viral sequences at the 5′ and 3′ ends of the molecule remain as single-stranded overhangs and mediate RNA cyclization by long-distance RNA–RNA contact (arrow). (B) A representative image showing individual molecules of purified NS5pol. (C) A representative image obtained with a sample containing both RNA and the viral NS5pol. The arrow indicates the polymerase bound to the RNA. Image sizes are 400 nm × 400 nm.
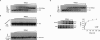
DV NS5pol binds to 5′ end sequences of the viral genome. (A) EMSA showing the interaction between the 5′DV RNA probe, corresponding to the first 160 nt of the DV genome (schematically represented on the left), and the purified NS5pol. Uniformly 32P-labeled 5′DV RNA (0.1 nM, 30,000 cpm) was titrated with increasing concentrations of NS5pol from 0 to 240 nM. (B) EMSA showing the lack of interaction between the 3′SL RNA probe corresponding to the last 106 nt of the DV genome and the purified NS5pol. (C) EMSA showing the lack of interaction between the 5′DV RNA probe with a deletion of SLA (5′DVΔSLA probe, left) and the purified NS5pol. (D) EMSA showing the interaction between SLA probe and purified NS5pol. The binding reactions were as described in A. (E) Interaction of NS5pol with the 5′DV and the SLA probes monitored by a filter-binding assay. Uniformly 32P-labeled RNA (0.1 nM) was incubated with increasing concentrations of NS5pol. (Left) RNA probes bound to each membrane as a function of NS5pol concentration visualized by PhosphorImaging. “Bound” indicates RNA–protein complexes retained in the nitrocellulose membrane, and “free” denotes the unbound probes retained in the nylon membrane. (Right) Quantification of the percentage of RNA probe bound was plotted as a function of NS5pol concentration, and Equation 1 was fitted.
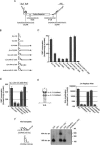
Role of long-range 5′–3′ RNA–RNA interactions during DV RNA synthesis. (A) Schematic representation of the DV genome showing the location and nucleotide sequence of the complementary regions 5′UAR, 5′CS, 3′CS, and 3′UAR. The locations of stem–loop A (SLA), stem–loop B (SLB), and 3′ stem–loop (3′SL) are also indicated. (B) Schematic representation of RNA molecules used as templates for the viral NS5pol RdRp activity. RNAs carrying the viral SLA followed by unrelated sequences of 100, 500, or 2000 nt are labeled SLA-100, SLA-500, and SLA-1000, respectively. Incorporation of the 5′CS, 5′UAR, or 5′CS-5′UAR in the SLA-2000 yielded the SLA-CS 2000, SLA-UAR 2000, or SLA-UAR-CS 2000 RNAs, respectively. The MutSLA UAR-CS 2000 corresponds to the respective RNA with a 4-nt substitution at the top of SLA (CAGA was replaced by AGAC). The white and black boxes shown at the 3′ of the molecules represent the 3′UAR and 3′CS regions, respectively. (C) In vitro RNA synthesis by the viral NS5pol using RNA templates described in B. The RdRp assay was carried out as described in Materials and Methods. (D) In vitro RNA synthesis by the viral NS5pol using the RNA template SLA-UAR CS 2000 described above, carrying specific mutations at 5′–3′UAR or 5′–3′CS regions that disrupted or reconstituted base-pairings. RNA mutants within 5′UAR or 5′CS (5′UARMut and 5′CSMut), which generated three and four mismatches when hybridized with the 3′UAR and 3′CS, respectively, and double mutants at the 5′ and 3′ regions (5′– 3′UARMut and 5′–3′CSMut RNAs), which compensated complementarity, were used as templates for polymerase activity. RdRp activity for the viral NS5pol was determined for each template and expressed as described in A. (E) DV replicon RNA amplification in BHK cells requires 5′–3′UAR complementarity. RNA synthesis of DV replicons with specific mutations within 5′UAR (5′UARMut1 and5′UARMut2, left) or double mutations at 5′ and 3′UAR (5′–3′UAR-Mut1 and 5′–3′UARMut2) that restored complementarity was evaluated by reporter activity 72 h after RNA transfections into BHK cells and compared with wild-type and replication-incompetent (MutNS5) replicons. Normalized luciferase levels were obtained using a control RNA encoding Renilla luciferase that was cotransfected with each replicon RNA. The normalized luciferase activity is shown in logarithmic scale. (F) SLA promotes RdRp activity of the viral NS5pol in trans. (Left) Schematic representations of the RNA templates used; (right) a denatured polyacrylamide gel showing the radiolabeled products from in vitro RdRp activity using NS5pol (6 ng/μL) and 300 nM of the templates described at the top of the gel. Arrows indicate the mobility of the full-size products of 454 and 160 nt. Mobilities of RNA markers (Mk) are also indicated on the right.
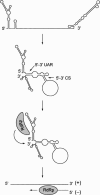
Model for DV minus strand RNA synthesis. The viral genome circularizes in the absence of proteins mediated by 5′– 3′ UAR and 5′–3′ CS hybridization. The viral RdRp binds to a 5′ stem–loop (SLA), and by long-range RNA–RNA interactions the polymerase is transferred to the site of initiation at the 3′ end of the genome.
Similar articles
-
Friedrich S, Engelmann S, Schmidt T, Szczepankiewicz G, Bergs S, Liebert UG, Kümmerer BM, Golbik RP, Behrens SE. Friedrich S, et al. J Virol. 2018 Feb 26;92(6):e01647-17. doi: 10.1128/JVI.01647-17. Print 2018 Mar 15. J Virol. 2018. PMID: 29263261 Free PMC article.
-
Functional RNA elements in the dengue virus genome.
Gebhard LG, Filomatori CV, Gamarnik AV. Gebhard LG, et al. Viruses. 2011 Sep;3(9):1739-56. doi: 10.3390/v3091739. Epub 2011 Sep 15. Viruses. 2011. PMID: 21994804 Free PMC article. Review.
-
Liu ZY, Li XF, Jiang T, Deng YQ, Ye Q, Zhao H, Yu JY, Qin CF. Liu ZY, et al. Elife. 2016 Oct 1;5:e17636. doi: 10.7554/eLife.17636. Elife. 2016. PMID: 27692070 Free PMC article.
-
Interactions between the Dengue Virus Polymerase NS5 and Stem-Loop A.
Bujalowski PJ, Bujalowski W, Choi KH. Bujalowski PJ, et al. J Virol. 2017 May 12;91(11):e00047-17. doi: 10.1128/JVI.00047-17. Print 2017 Jun 1. J Virol. 2017. PMID: 28356528 Free PMC article.
-
Dynamic RNA structures in the dengue virus genome.
Iglesias NG, Gamarnik AV. Iglesias NG, et al. RNA Biol. 2011 Mar-Apr;8(2):249-57. doi: 10.4161/rna.8.2.14992. Epub 2011 Mar 1. RNA Biol. 2011. PMID: 21593583 Review.
Cited by
-
Bujalowski PJ, Bujalowski W, Choi KH. Bujalowski PJ, et al. Sci Rep. 2020 Aug 6;10(1):13306. doi: 10.1038/s41598-020-70094-y. Sci Rep. 2020. PMID: 32764551 Free PMC article.
-
A New Subclass of Exoribonuclease-Resistant RNA Found in Multiple Genera of Flaviviridae.
Szucs MJ, Nichols PJ, Jones RA, Vicens Q, Kieft JS. Szucs MJ, et al. mBio. 2020 Sep 29;11(5):e02352-20. doi: 10.1128/mBio.02352-20. mBio. 2020. PMID: 32994331 Free PMC article.
-
Wastika CE, Harima H, Sasaki M, Hang'ombe BM, Eshita Y, Qiu Y, Hall WW, Wolfinger MT, Sawa H, Orba Y. Wastika CE, et al. Viruses. 2020 Sep 11;12(9):1017. doi: 10.3390/v12091017. Viruses. 2020. PMID: 32933075 Free PMC article.
-
Baker C, Xie X, Zou J, Muruato A, Fink K, Shi PY. Baker C, et al. EBioMedicine. 2020 Jul;57:102838. doi: 10.1016/j.ebiom.2020.102838. Epub 2020 Jun 20. EBioMedicine. 2020. PMID: 32574959 Free PMC article.
-
Regulation of flavivirus RNA synthesis and capping.
Saeedi BJ, Geiss BJ. Saeedi BJ, et al. Wiley Interdiscip Rev RNA. 2013 Nov-Dec;4(6):723-35. doi: 10.1002/wrna.1191. Epub 2013 Aug 8. Wiley Interdiscip Rev RNA. 2013. PMID: 23929625 Free PMC article. Review.
References
-
- Ackermann M., Padmanabhan R. De novo synthesis of RNA by the dengue virus RNA-dependent RNA polymer-ase exhibits temperature dependence at the initiation but not elongation phase. J. Biol. Chem. 2001;276:39926–39937. - PubMed
-
- Alvarez D.E., De Lella Ezcurra A.L., Fucito S., Gamarnik A.V. Role of RNA structures present at the 3′UTR of dengue virus on translation, RNA synthesis, and viral replication. Virology. 2005a;339:200–212. - PubMed
-
- Andino R., Rieckhof G.E., Baltimore D. A functional ribonucleoprotein complex forms around the 5′ end of poliovirus RNA. Cell. 1990;63:369–380. - PubMed
-
- Brinton M.A., Fernandez A.V., Dispoto J.H. The 3′-nucleotides of flavivirus genomic RNA form a conserved secondary structure. Virology. 1986;153:113–121. - PubMed
Publication types
MeSH terms
Substances
LinkOut - more resources
Full Text Sources
Other Literature Sources
Research Materials