Apicoplast fatty acid synthesis is essential for organelle biogenesis and parasite survival in Toxoplasma gondii - PubMed
- ️Sun Jan 01 2006
Apicoplast fatty acid synthesis is essential for organelle biogenesis and parasite survival in Toxoplasma gondii
Jolly Mazumdar et al. Proc Natl Acad Sci U S A. 2006.
Abstract
Apicomplexan parasites are the cause of numerous important human diseases including malaria and AIDS-associated opportunistic infections. Drug treatment for these diseases is not satisfactory and is threatened by resistance. The discovery of the apicoplast, a chloroplast-like organelle, presents drug targets unique to these parasites. The apicoplast-localized fatty acid synthesis (FAS II) pathway, a metabolic process fundamentally divergent from the analogous FAS I pathway in humans, represents one such target. However, the specific biological roles of apicoplast FAS II remain elusive. Furthermore, the parasite genome encodes additional and potentially redundant pathways for the synthesis of fatty acids. We have constructed a conditional null mutant of acyl carrier protein, a central component of the FAS II pathway in Toxoplasma gondii. Loss of FAS II severely compromises parasite growth in culture. We show FAS II to be required for the activation of pyruvate dehydrogenase, an important source of the metabolic precursor acetyl-CoA. Interestingly, acyl carrier protein knockout also leads to defects in apicoplast biogenesis and a consequent loss of the organelle. Most importantly, in vivo knockdown of apicoplast FAS II in a mouse model results in cure from a lethal challenge infection. In conclusion, our study demonstrates a direct link between apicoplast FAS II functions and parasite survival and pathogenesis. Our genetic model also offers a platform to dissect the integration of the apicoplast into parasite metabolism, especially its postulated interaction with the mitochondrion.
Conflict of interest statement
Conflict of interest statement: No conflicts declared.
Figures
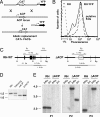
Gene targeting of the ACP locus using a positive/negative selection scheme to enrich homologous recombinants. (A) Schematic representation of the two-marker targeting plasmid and positive/negative selection for homologous recombination at the ACP locus. (B) FACS profiles of the nonfluorescent wild type (RH, short-dashed line), a YFP-expressing clone derived from this strain (long-dashed line), and a population of stable drug-resistant parasites after transfection with the double-marker targeting plasmid (solid line, putative KOs; the indicated 1% least fluorescent of parasites were sorted and cloned). Two of 25 of these clones showed successful targeting of the locus (compared with >400 clones unsuccessfully screened by using a single-marker approach). (C) Allelic replacement of the native ACP gene by chloramphenicol acetyl transferase (CAT) gene yields altered restriction profile after digestion with restriction enzymes BamHI, BglII, and XhoI. (D) PCR detection of endogenous (1.5 kb) and inducible (1.3 kb) ACP genes. Heterolog., clone harboring a heterologous insertion of the targeting plasmid in addition to the native locus. The pKO targeting plasmid and RH genomic DNA serve as control for KO insert and native ACP, respectively. (E) Southern blot analysis of BamHI/BglII and BamHI/XhoI digests of RH and ΔACP/ACPi genomic DNA with probes P1 (ACP intron, hybridizes to native ACP but not to the ectopic minigene copy), P2 (CAT coding sequence), and P3 (3′ noncoding region present in both loci). All autoradiographs show the same membrane, which was stripped and reprobed.
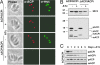
Regulation of ACP expression by ATc treatment. (A) ACP/ACPi and ΔACP/ACPi parasites were treated with ATc for 5 days and fixed and stained with antibodies to ACP and c-myc (same exposure time for all micrographs). (B) Western blot analysis of the same set of samples using ACP antibody. p, precursor; m, mature protein; MIC2, loading control. (C) ACPi parasites were grown in the presence of ATc, and samples were collected daily for 5 days and analyzed by Western blot by using the ACP antibody (native ACP serves as loading control). Note that the precursor protein disappears within a day, suggesting fast suppression of transcription, whereas the mature protein is stable and has to be diluted by growth.
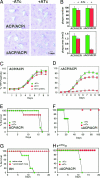
Growth and virulence of mutant and parental strain. (A) Growth of ACP/ACPi and ΔACP/ACPi parasites incubated in the presence and absence of ATc was scored by plaque assay. (B) Plaque number and area were quantified in three independent flasks. (C and D) ATc dependence of growth was also measured by following fluorescence in YFP-YFP-expressing lines derived from parent and mutant (second-passage parasites were cultured for 6 days under ATc before inoculation of multiwell plates). C57BL/6 mice were infected with parent (E) and mutant (F) parasites (note slight delay in killing by the mutant when compared with the parental parasites). Mice received 0.2 mg/ml ATc (red) or placebo (green) in the drinking water, and survival was followed for 100 days. (G) Surviving mice from F were rechallenged with 10,000 RH wild-type parasites (vaccinated parasites) in parallel to a group of naïve control mice. (H) Mice were immunosuppressed by injection of 0.5 mg of an IFNγ-specific antibody on days 0 and 5 (arrows), infected with ΔACP/ACPi, and treated as described for F.
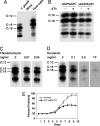
14C lack of apicoplast FAS II does not affect acetate incorporation but blocks lipoylation of apicoplast PDH. (A) Extracellular tachyzoites were metabolically labeled with [14C]acetate. Lipids were extracted and hydrolyzed, and the resulting fatty acids were methylated. Radiolabeled fatty acid methyl esters were analyzed by reversed-phase high-performance thin-layer chromatography (methyl esters of radiolabeled fatty acid of known chain length were run in parallel as standards). Mock infection or direct labeling of host cell cultures served as control for potential host cell contamination. (B) ΔACP/ACPi and ACP/ACPi parasites were grown in the presence or absence of ATc for 6 days followed before [14C]acetate labeling. (C and D) Parasite acetate incorporation into fatty acids was resistant to the FAS II inhibitor thiolactomycin (C) but sensitive to the general FAS inhibitor cerulenin (D). (E) Growth of the ΔACP/ACPi-YFP-YFP strain was measured in the presence of 0.01 mg/ml thiolactomycin (filled squares) in comparison with untreated controls (open circles). Thiolactomycin showed strong growth inhibition, and [14C]acetate incorporation was resistant to a 5-fold-higher drug concentration (C).
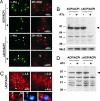
Loss of apicoplast FAS II abolishes lipoylation of apicoplast but not mitochondrial enzymes. (A and B) Immunofluorescence (A) and Western blot (B) analysis of parent and mutant cells with antibody to lipoylated PDH-E2 (2H-4C8). ATc treatment of the mutant abolishes PDH labeling (arrow). An antibody to the T. gondii apicoplast histone-like protein (Hu protein; S. Vaishnava and B.S., unpublished observations) served as apicoplast marker, and an antibody to MIC2 served as loading control. (C and D) Immunofluorescence (C) and Western blot (D) analysis using a polyclonal serum reactive to all lipoylated enzymes localized to the plastid (P) and the mitochondrion (M). Note loss of PDH labeling (black arrowhead) and persistence of mitochondrial labeling (white arrowhead).
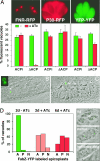
Depletion of ACP leads to defects in apicoplast morphology and biogenesis. (A) ACP/ACPi and ΔACP/ACPi cells were grown for 6 days in the presence or absence of ATc and then transiently transfected with plasmids, resulting in the expression of FNR-RFP (apicoplast), P30-RFP (dense granules and parasitophorous vacuole), and YFP-YFP (cytoplasm) before infection of coverslip cultures. After 24 h parasites were scored for fluorescent protein expression. (B and C) A ΔACP/ACPi line stably expressing the apicoplast marker FabZ-YFP was treated with ATc for 3 days and imaged in vivo. Vacuoles with a single or a few large apicoplasts were frequently observed (arrowhead; parasitophorous vacuoles are indicated by dotted lines). (D) The same line was treated with ATc for 0, 2, and 6 days, and plastid morphology was scored by using the three categories indicated (A, all parasites in a vacuole show a fluorescent apicoplast; P, one or few apicoplasts per vacuole; N, no apicoplast labeling detected).
Similar articles
-
Bisanz C, Bastien O, Grando D, Jouhet J, Maréchal E, Cesbron-Delauw MF. Bisanz C, et al. Biochem J. 2006 Feb 15;394(Pt 1):197-205. doi: 10.1042/BJ20050609. Biochem J. 2006. PMID: 16246004 Free PMC article.
-
Martins-Duarte ÉS, Carias M, Vommaro R, Surolia N, de Souza W. Martins-Duarte ÉS, et al. J Cell Sci. 2016 Sep 1;129(17):3320-31. doi: 10.1242/jcs.185223. Epub 2016 Jul 25. J Cell Sci. 2016. PMID: 27457282
-
Fellows JD, Cipriano MJ, Agrawal S, Striepen B. Fellows JD, et al. mBio. 2017 Jun 27;8(3):e00950-17. doi: 10.1128/mBio.00950-17. mBio. 2017. PMID: 28655825 Free PMC article.
-
Fatty acid biosynthesis as a drug target in apicomplexan parasites.
Goodman CD, McFadden GI. Goodman CD, et al. Curr Drug Targets. 2007 Jan;8(1):15-30. doi: 10.2174/138945007779315579. Curr Drug Targets. 2007. PMID: 17266528 Review.
-
Seeber F. Seeber F. Curr Drug Targets Immune Endocr Metabol Disord. 2003 Jun;3(2):99-109. doi: 10.2174/1568008033340261. Curr Drug Targets Immune Endocr Metabol Disord. 2003. PMID: 12769782 Review.
Cited by
-
Agrawal S, Chung DW, Ponts N, van Dooren GG, Prudhomme J, Brooks CF, Rodrigues EM, Tan JC, Ferdig MT, Striepen B, Le Roch KG. Agrawal S, et al. PLoS Pathog. 2013;9(6):e1003426. doi: 10.1371/journal.ppat.1003426. Epub 2013 Jun 13. PLoS Pathog. 2013. PMID: 23785288 Free PMC article.
-
Parsons M, Karnataki A, Feagin JE, DeRocher A. Parsons M, et al. Eukaryot Cell. 2007 Jul;6(7):1081-8. doi: 10.1128/EC.00102-07. Epub 2007 May 18. Eukaryot Cell. 2007. PMID: 17513565 Free PMC article. Review. No abstract available.
-
Direct Effect of Two Naphthalene-Sulfonyl-Indole Compounds on Toxoplasma gondii Tachyzoite.
Asgari Q, Keshavarz H, Rezaeian M, Motazedian MH, Shojaee S, Mohebali M, Miri R. Asgari Q, et al. J Parasitol Res. 2013;2013:716976. doi: 10.1155/2013/716976. Epub 2013 Oct 21. J Parasitol Res. 2013. PMID: 24228173 Free PMC article.
-
Xu XP, Elsheikha HM, Liu WG, Zhang ZW, Sun LX, Liang QL, Song MX, Zhu XQ. Xu XP, et al. Front Microbiol. 2021 Aug 31;12:703059. doi: 10.3389/fmicb.2021.703059. eCollection 2021. Front Microbiol. 2021. PMID: 34531837 Free PMC article.
References
-
- Kohler S., Delwiche C. F., Denny P. W., Tilney L. G., Webster P., Wilson R. J., Palmer J. D., Roos D. S. Science. 1997;275:1485–1489. - PubMed
-
- McFadden G. I., Reith M. E., Munholland J., Lang-Unnasch N. Nature. 1996;381:482. - PubMed
-
- Ralph S. A., Van Dooren G. G., Waller R. F., Crawford M. J., Fraunholz M. J., Foth B. J., Tonkin C. J., Roos D. S., McFadden G. I. Nat. Rev. Microbiol. 2004;2:203–216. - PubMed
-
- McFadden G. I., Roos D. S. Trends Microbiol. 1999;7:328–333. - PubMed
Publication types
MeSH terms
Substances
Grants and funding
LinkOut - more resources
Full Text Sources
Other Literature Sources
Research Materials
Miscellaneous