Crystal structure of SV40 large T-antigen bound to p53: interplay between a viral oncoprotein and a cellular tumor suppressor - PubMed
- ️Sun Jan 01 2006
Crystal structure of SV40 large T-antigen bound to p53: interplay between a viral oncoprotein and a cellular tumor suppressor
Wayne Lilyestrom et al. Genes Dev. 2006.
Abstract
The transformation potential of Simian Virus 40 depends on the activities of large T-antigen (LTag), which interacts with several cellular tumor suppressors including the important "guardian" of the genome, p53. Inhibition of p53 function by LTag is necessary for both efficient viral replication and cellular transformation. We determined the crystal structure of LTag in complex with p53. The structure reveals an unexpected hexameric complex of LTag binding six p53 monomers. Structure-guided mutagenesis of LTag and p53 residues supported the p53-LTag interface defined by the complex structure. The structure also shows that LTag binding induces dramatic conformational changes at the DNA-binding area of p53, which is achieved partially through an unusual "methionine switch" within p53. In the complex structure, LTag occupies the whole p53 DNA-binding surface and likely interferes with formation of a functional p53 tetramer. In addition, we showed that p53 inhibited LTag helicase function through direct complex formation.
Figures
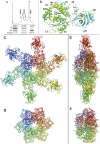
Overall structure of LTag–p53 complex. (A) The gel filtration profile of the LTag–p53 complex. The protein complex was stable when analyzed by size-exclusion chromatography on a superdex200 column. The positions for the molecular weight standard markers are indicated. Peak 1 contained LTag–p53 complex in a hexameric form (~360 kDa), peak 2 contained LTag–p53 complex in a monomeric form (~69 kDa), and peak 3 contained p53 alone (~22 kDa). The three SDS-PAGE gels below the profile show the presence of LTag and p53 in the three peaks. The excess of p53 was eluted in peak 3. (B) The monomer structure of LTag (in green) and p53 (in cyan). In order to better display the interface, each molecule was rotated 60° from its original position within the complex. The regions involved in the interface contacts in the complex are colored in yellow. LTag domains D1, D2, and D3 are indicated. (C,D) The top views of the LTag hexamer in complex with p53 (C) and the LTag hexamer stripping off p53 (D). Each of the six subunits of a LTag hexamer binds one p53 molecule as shown in A. (E,F) The side views of the LTag hexamer in complex with p53 (E) and the hexamer stripping of the p53 (F). The side view of the LTag–p53 complex structure reveals that p53 binds to the larger C-terminal tier of LTag.
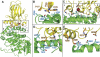
Residues involved in LTag–p53 interactions. (A) A ribbon illustration of a monomeric LTag–p53 complex taken from the hexameric structure. The boxes indicate the two regions at the interface between p53 (in yellow) and LTag (in green). (B) The close-up view of the interactions in box 1, showing that p53 residues P177 and H178 make van der Waals contacts with LTag residues A586 and V597, respectively, and p53 R181 bonds with LTag Q593. (C) The LTag–p53 interface around the zinc motif of p53. The zinc atom is between the two boxed regions, bridging and stabilizing p53 surface loops that contact LTag. (D,E) The close-up views of the detailed interactions within. D shows a hydrophobic pocket formed by LTag residues L609, W581, Y582, and Y612, with which p53 M246 interacts. E shows that four bonds form between p53 N288, R273, N239, and R280 with LTag residues Q613, D604, E601, and D402, respectively.
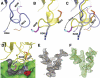
The conformational change of p53 upon LTag binding. (A,B) The L3 conformation of p53 in the DNA-binding or LTag-binding state. (C) Superposition of the p53 Ca carbon, showing the L3 conformation changes upon LTag binding. The LTag-binding state of p53 is shown with a yellow backbone and thick side chains, and the DNA-binding state with a light-blue backbone and thin side chain. Arrows indicate the movement of the three key residues upon LTag binding. The originally buried M246 residue in the DNA-binding state flips out from the interior core to extend and insert its side chain into the hydrophobic pocket on LTag as shown in D. At the same time, the void left by the flipped M246 is filled by another residue, M243, that is flipped inside from its exposed position in the DNA-binding state. This “methionine switch” allows L3 to have a large conformational change. (D) Interactions of p53 with LTag through the L3 loop. The flipped-out M246 on the L3 loop extends and inserts into the hydrophobic pocket on LTag, while R248 establishes a salt bridge on the surface (also see Fig. 2D). (E,F) The 12-fold averaged electron density map and the simulated annealing omit map at the position of the L3 loop of p53 in the LTag complex structure.
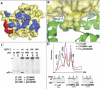
Mutational analysis at the LTag–p53 interface. (A) The surface representation of p53, showing locations of the DNA-binding and LTag-binding residues. The DNA-binding residues are colored in red, the LTag-binding residues in blue, and residues involved in both DNA binding and LTag binding are colored in silver. The figure shows that p53–DNA interaction residues are adjacent and overlapping with the p53–LTag interface. The binding of LTag to these p53 residues can effectively shield the entire DNA-binding surface of p53, which seems a very reliable mechanism for inhibiting transactivation at the p53-dependent promoters. Interestingly, the three most commonly mutated p53 residues in cancer—R273, R248, and G245 (indicated by *)—are also recognized by LTag. (B) The positions of the three residues at the LTag–p53 interface; that is, LTag residues V585 and D604 (in cyan) and p53 residue M246 (in orange), which are targeted for mutational analysis to verify the structure. The structure shows that these residues contribute significantly to the binding of LTag and p53, and thus mutations of these residues are expected to disrupt LTag–p53 interactions. (C) Formation of LTag–p53 complex using the LTag or p53 mutants, as assayed by the GST pull-down method. GST-LT fusion proteins (both wild-type and mutant LTag) bound on the glutathione resin were used to test its ability to bind to wild-type and mutant p53 proteins on the column. Lanes 1 and 2 are controls for nonspecific binding of wild-type (wt) or mutant p53 proteins alone to the glutathione resin, respectively, lane 3 is the wild-type GST-LT pulling down wild-type p53 on the glutathione resin, lane 4 is the wild-type GST-LT pulling-down mutant p53 M246R (M/R), lane 5 is the mutant GST-LT V585R (V/R) pulling down wild-type p53, and lane 6 is the mutant GST-LT D604R (D/R) pulling down wild-type p53. The result shows that LTag mutants V585R and D604R and the p53 mutant M246R all disrupted the LTag–p53 complex formation. (D) Effects of structure-based mutations on LTag–p53 complex formation, as assayed by size-exclusion chromatography. The various mutants and wild-type protein mixtures of LTag and p53 were incubated for 30 min at 25°C prior to loading on a superdex200 analytical gel filtration column. The positions of the molecular weight markers are indicated on the chromatograph. Fractions corresponding to the three elution peaks (peaks 1, 2, and 3) for each of the experiments were analyzed using SDS-PAGE; the results are shown in the panel below the gel filtration chromatograph. The elution volumes equivalent to peaks 1 and 2, where the hexameric and nonhexameric complexes of LTag–p53 normally migrate, contained very little p53 when either mutant LTag or mutant p53 was used to form the complex with the corresponding wild-type partner. Both the affinity-binding studies (shown in C) and the gel filtration analyses indicated that the structure-based mutations have the desired effect disrupting the interactions between LTag and p53.
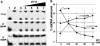
Effect of p53 binding on LTag helicase activity. (A) The helicase activity of wild-type (wt) and mutant LTag was assayed in the presence of varying amounts of p53. The LTag mutants V585R and D604R used in this assay are defective in binding to p53. For each gel on this panel, lanes 1 and 2 are the boiled and unboiled dsDNA substrate alone. Lanes 3–7 contain 20 nM wild-type or mutant LTag proteins. The p53 concentrations are as follows: 0 nM (lanes 1–3); 12.5 nM (lane 4); 25 nM (lane 5); 50 nM (lane 6); 75 nM (lane 7). (B) A plot summarizing the results of the helicase as-says shown in A. The helicase activity of wild-type LTag was proportionally inhibited by higher concentrations of p53. In contrast, the two LTag mutants that are defective in p53 binding were insensitive to even the highest concentrations of p53 tested.
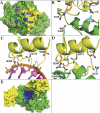
Mapping of LTag mutations defective in transformation and p53 binding. (A) Mapping of LTag mutations that disrupt transformation (colored in blue and purple) on the LTag surface. These residues are located near or within the p53-binding surface (colored in yellow). (B) Interactions with p53 (in yellow and orange) through two of the LTag residues (D402 and P584, in green and cyan) critical for cell transformation by LTag. D402 is involved in a salt bridge with R248 of p53; residue P584 makes van der Waals contact with M243 of p53. (C,D) DNA mimicry by LTag in one of the interface areas, where p53 (in yellow) uses the same set of side chains (mostly from h2, residues R273, R280, N288, and N239) to bond with DNA in C or LTag in D. (E) Surface rendering of p53 (in yellow) and LTag (in green) complex, showing the bound p53 is adjacent to LTag's side-channel opening (in blue).
Comment in
-
When viral oncoprotein meets tumor suppressor: a structural view.
Liu X, Marmorstein R. Liu X, et al. Genes Dev. 2006 Sep 1;20(17):2332-7. doi: 10.1101/gad.1471706. Genes Dev. 2006. PMID: 16951249 No abstract available.
Similar articles
-
Structure of the replicative helicase of the oncoprotein SV40 large tumour antigen.
Li D, Zhao R, Lilyestrom W, Gai D, Zhang R, DeCaprio JA, Fanning E, Jochimiak A, Szakonyi G, Chen XS. Li D, et al. Nature. 2003 May 29;423(6939):512-8. doi: 10.1038/nature01691. Nature. 2003. PMID: 12774115
-
A computational analysis of ATP binding of SV40 large tumor antigen helicase motor.
Shi Y, Liu H, Gai D, Ma J, Chen XS. Shi Y, et al. PLoS Comput Biol. 2009 Sep;5(9):e1000514. doi: 10.1371/journal.pcbi.1000514. Epub 2009 Sep 25. PLoS Comput Biol. 2009. PMID: 19779548 Free PMC article.
-
Gai D, Zhao R, Li D, Finkielstein CV, Chen XS. Gai D, et al. Cell. 2004 Oct 1;119(1):47-60. doi: 10.1016/j.cell.2004.09.017. Cell. 2004. PMID: 15454080
-
Interactions between SV40 large-tumor antigen and the growth suppressor proteins pRB and p53.
Ludlow JW. Ludlow JW. FASEB J. 1993 Jul;7(10):866-71. doi: 10.1096/fasebj.7.10.8344486. FASEB J. 1993. PMID: 8344486 Review.
-
The role of the J domain of SV40 large T in cellular transformation.
DeCaprio JA. DeCaprio JA. Biologicals. 1999 Mar;27(1):23-8. doi: 10.1006/biol.1998.0173. Biologicals. 1999. PMID: 10441399 Review.
Cited by
-
Yeager C, Carter G, Gohara DW, Yennawar NH, Enemark EJ, Arnold JJ, Cameron CE. Yeager C, et al. Nucleic Acids Res. 2022 Nov 11;50(20):11775-11798. doi: 10.1093/nar/gkac1054. Nucleic Acids Res. 2022. PMID: 36399514 Free PMC article.
-
The Role of the Large T Antigen in the Molecular Pathogenesis of Merkel Cell Carcinoma.
Myrda J, Bremm F, Schaft N, Dörrie J. Myrda J, et al. Genes (Basel). 2024 Aug 27;15(9):1127. doi: 10.3390/genes15091127. Genes (Basel). 2024. PMID: 39336718 Free PMC article. Review.
-
The crystal structure of the SV40 T-antigen origin binding domain in complex with DNA.
Meinke G, Phelan P, Moine S, Bochkareva E, Bochkarev A, Bullock PA, Bohm A. Meinke G, et al. PLoS Biol. 2007 Feb;5(2):e23. doi: 10.1371/journal.pbio.0050023. PLoS Biol. 2007. PMID: 17253903 Free PMC article.
-
Ou HD, May AP, O'Shea CC. Ou HD, et al. Wiley Interdiscip Rev Syst Biol Med. 2011 Jan-Feb;3(1):48-73. doi: 10.1002/wsbm.88. Wiley Interdiscip Rev Syst Biol Med. 2011. PMID: 21061422 Free PMC article. Review.
-
A two-hybrid assay to study protein interactions within the secretory pathway.
Dube DH, Li B, Greenblatt EJ, Nimer S, Raymond AK, Kohler JJ. Dube DH, et al. PLoS One. 2010 Dec 28;5(12):e15648. doi: 10.1371/journal.pone.0015648. PLoS One. 2010. PMID: 21209940 Free PMC article.
References
-
- Bargonetti J., Reynisdottir I., Friedman P.N., Prives C. Site-specific binding of wild-type p53 to cellular DNA is inhibited by SV40 T antigen and mutant p53. Genes & Dev. 1992;6:1886–1898. - PubMed
-
- Brunger A.T., Adams P.D., Clore G.M., DeLano W.L., Gros P., Grosse-Kunstleve R.W., Jiang J.S., Kuszewski J., Nilges M., Pannu N.S., et al. Crystallography and NMR system: A new software suite for macromolecular structure determination. Acta Crystallogr. D Biol. Crystallogr. 1998;54((Pt5)):905–921. - PubMed
-
- Cho Y., Gorina S., Jeffrey P.D., Pavletich N.P. Crystal structure of a p53 tumor suppressor–DNA complex: Understanding tumorigenic mutations. Science. 1994;265:346–355. - PubMed
Publication types
MeSH terms
Substances
LinkOut - more resources
Full Text Sources
Other Literature Sources
Molecular Biology Databases
Research Materials
Miscellaneous