Inositol monophosphatase regulates localization of synaptic components and behavior in the mature nervous system of C. elegans - PubMed
- ️Sun Jan 01 2006
Inositol monophosphatase regulates localization of synaptic components and behavior in the mature nervous system of C. elegans
Yoshinori Tanizawa et al. Genes Dev. 2006.
Abstract
Although recent studies have provided significant molecular insights into the establishment of neuronal polarity in vitro, evidence is lacking on the corresponding phenomena in vivo, including correct localization of synaptic components and the importance of this process for function of the nervous system as a whole. RIA interneurons act as a pivotal component of the neural circuit for thermotaxis behavior in the nematode Caenorhabditis elegans and provide a suitable model to investigate these issues, having a neurite clearly divided into pre- and post-synaptic regions. In a screen for thermotaxis mutants, we identified the gene ttx-7, which encodes myo-inositol monophosphatase (IMPase), an inositol-producing enzyme regarded as a bipolar disorder-relevant molecule for its lithium sensitivity. Here we show that mutations in ttx-7 cause defects in thermotaxis behavior and localization of synaptic proteins in RIA neurons in vivo. Both behavioral and localization defects in ttx-7 mutants were rescued by expression of IMPase in adults and by inositol application, and the same defects were mimicked by lithium treatment in wild-type animals. These results suggest that IMPase is required in central interneurons of the mature nervous system for correct localization of synaptic components and thus for normal behavior.
Figures
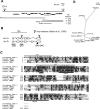
Genetic and molecular analyses of ttx-7. (A) Position of ttx-7 on chromosome I. ttx-7 was mapped between two SNPs. Results of rescue experiments for ttx-7 mutant thermotaxis defects are indicated as + (rescued) or − (not rescued). The numbers in parentheses are the fraction of rescued lines. (B) Two types of cDNA clones. Exons are boxed, and key residues are indicated by open triangles (Atack et al. 1995). Mutation sites of ttx-7 mutants are illustrated. (C) Comparison of sequences of TTX-7A and IMPases in other species. Black and gray boxes highlight identical and similar residues, respectively. Black bars below the sequences indicate conserved motifs, and asterisks indicate key residues (Atack et al. 1995). The aspartate with a white asterisk is the residue whose conversion to asparagine abolished the enzymatic activity of TTX-7 (see text). Deletions are indicated by gray bars above the sequences. (D) Phylogenic analysis of full-length sequences of IMPase orthologs and two other C. elegans proteins, ZK430.2 and Y6B3B.5, that have less similarity to human IMPases than TTX-7 (Supplementary Table S2). The dendrogram was generated with ClustalW and NJplot.
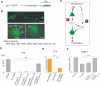
Expression pattern and cell-autonomous function of TTX-7 in RIA neurons. (A) The ttx-7∷EGFP fusion gene. (B) Expression of ttx-7∷EGFP. Anterior is to the left, dorsal is up. (Top panel) Strong fluorescence was observed in head and tail neurons (arrow) and coelomocytes (arrowhead). Bar, 100 μm. (Bottom left panel) In the head, a limited number of neurons express ttx-7∷EGFP. Bar, 5 μm. (Bottom right panel) Expression in RIA neurons was observed in another focal plane. Bar, 5 μm. Other identified neurons are listed in the figure. (C) The proposed thermotaxis neural circuit (Mori and Ohshima 1995). AFD is a major thermosensory neuron, and X is a putative minor thermosensory neuron. AFD and AIY neurons promote thermophilic behavior (indicated as “T”), and AIZ neurons promote cryophilic behavior (indicated as “C”). RIA neurons are proposed to integrate these signals. In this circuit, AFD and RIA express ttx-7 and are colored green. (D) Cell-specific rescue of the thermotaxis defect in ttx-7 mutants by ttx-7a cDNA expression. Bars show the percentage of animals that moved to the cultivation temperature (20°C). osm-6p induces expression in many sensory neurons. Compared with naïve ttx-7 mutants, only transgenic strains with RIAp∷ttx-7a showed improved thermotaxis (Dunnett’s multiple comparison test; n = 3 or more assays). (E) Genetic epistasis of ttx-7 and tax-6 mutants in thermotaxis. Note that the bars indicate the percentage of thermophilic animals. The ttx-7 mutation suppressed the thermophilic behavior of tax-6 mutants, but the double mutants were still more thermophilic than ttx-7 mutants (Dunnett’s multiple comparison test; n = 4 or more assays). (F) Cell-specific rescue of the SNB-1 localization defect in ttx-7 mutants. Localization indexes were calculated as shown in Figure 3B and Materials and Methods. The localization was rescued not with AIYp∷ttx-7a but with RIAp∷ttx-7a (Steel’s multiple comparison test; n = 10 animals).
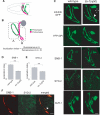
Synaptic defects in RIA neurons. (A) Schematic drawing of the head region. Colored circles and lines represent cell bodies and neurites of RIA neurons, respectively. Left (green) and right (magenta) counterparts, whose neurites overlap in their distal regions, are illustrated. Anterior is to the left, dorsal is up. (B) Schematic drawing of synapse distribution in an RIA neuron (White et al. 1986), and calculation of the localization index. Presynapses and post-synapses are indicated in green and magenta, respectively. Fluorescence intensity in regions A (distal neurite) and B (isthmus and proximal neurite) are measured and used to calculate the localization index with the formula described in Materials and Methods. A single RIA neuron is illustrated for simplicity. (C) Fluorescence images of RIA neurons in wild-type and ttx-7 animals. (First row) RIA neurons expressing soluble GFP. In ttx-7 mutants, minor swellings in the neurite were observed more often than in wild type (arrowhead). (Second row) RIA neurons expressing YFP-GPI. (Third row) RIA neurons expressing SNB-1∷VENUS. (Fourth row) RIA neurons expressing GFP∷SYD-2. (Fifth row) RIA neurons expressing GLR-1∷GFP. Bar, 5 μm. (D) Localization indexes of SNB-1∷VENUS. ttx-7 mutants showed a lower index than wild-type animals (Mann-Whitney U-test; n = 11 for wild type, n = 10 for ttx-7). (E) Localization indexes of GFP∷SYD-2 are also lower in ttx-7 mutants than in wild-type animals (Mann-Whitney U-test) (n = 20 animals). (F) Localization of SNB-1∷ECFP (left) and GFP∷SYD-2 (middle) in ttx-7 mutants were simultaneously visualized. (Right panel) Both were often observed to accumulate in the distal end of the post-synaptic region (arrowhead). Dorsal is up, anterior is to the left. Bar, 5 μm.
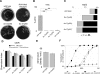
Behavioral defects in ttx-7 mutants. (A) Thermotaxis tracks of naïve wild-type, RIA-killed wild-type, and ttx-7 animals after cultivation at 20°C. A radial thermal gradient was established on the assay plates, which was 17°C at the center and 25°C at the periphery. (B) Thermotaxis assay. Bars indicate the percentage of animals that moved to the cultivation temperature, 20°C, on the assay plates (n ≥ 4 assays). Dunnett’s multiple comparison test was performed. (C) Chemotaxis to the soluble attractant NaCl. (+) Strong attraction; (+/−) moderate attraction; (−) no attraction. No difference between wild type and ttx-7 mutants was observed. n = 60 animals. (D) Chemotaxis to volatile attractants. Bars show the chemotaxis index indicating the extent of attraction, which did not differ significantly between wild type and ttx-7. n = 3 or more assays. (E) Bend counts per minute did not differ significantly between wild type and ttx-7 (Mann-Whitney U-test; n = 10 animals). (F) Sensitivity to aldicarb (1 mM). The graph shows the percentage of animals paralyzed at the time indicated. The EC50 values calculated with Origin software did not differ significantly between wild-type and ttx-7 animals (Student’s t-test; n = 3 assays, 25 animals assayed per strain).
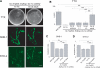
Rescue of defects by adult-stage-specific expression of ttx-7. (A) Thermotaxis tracks and synaptic protein localization in ttx-7 mutants with transgene hsp16-2p∷ttx-7a cDNA. Animals had not been (no hs) or had been (hs at adult) exposed to heat shock at the adult stage. (B) Heat-shock rescue of thermotaxis. ttx-7 mutants with the transgene were exposed to heat shock at the stages indicated and were assayed as adults. ttx-7 mutants without the transgene were not rescued by heat shock (data not shown) (Dunnett’s multiple comparison test; n = 3 assays). (C) Localization indexes of SNB-1∷VENUS in heat-shock experiments. TTX-7 expression in adult stages rescued SNB-1 localization, although the index of rescued animals was still lower than that of wild-type animals (Steel’s multiple comparison test; n = 10 animals). (D) Localization indexes of GFP∷SYD-2 in heat-shock experiments. TTX-7 expression at the adult stage rescued SYD-2 localization in ttx-7 mutants (Steel’s multiple comparison test; n = 10–20 animals).
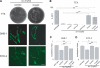
Effects of exogenously applied inositol. (A) Thermotaxis tracks and protein localization in RIA neurons of ttx-7 mutants grown from birth with sorbitol or inositol. (B) Effects of inositol and other monosaccharides on thermotaxis behavior of ttx-7 mutant animals. “Inositol at adult” indicates that animals were treated with inositol after they grew to be adults, whereas animals in the other conditions were cultivated under constant conditions (see text). Although not illustrated for simplicity, the difference between wild-type and the other groups, and the difference between each of the two right-most treatments and each of sorbitol, glucose, and galactose treatments, were also significant (p < 0.01, Tukey’s multiple comparison test; n ≥ 3 assays). (C,D) Localization index of SNB-1∷VENUS and GFP∷SYD-2 in animals grown with monosaccharides. Inositol application in the adult stage rescued the indexes of ttx-7 mutants, which were still lower than that of wild-type animals (Steel-Dwass multiple comparison test; n = 10 or more animals).
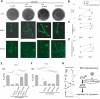
Effects of exogenously applied LiCl. (A) LiCl reversibly disrupts thermotaxis and protein localization. (First row) Thermotaxis. (Second row) SNB-1 localization. (Third row) SYD-2 localization. In each row, wild-type animals that were cultivated without LiCl, (first column) with LiCl from birth (second column), and with LiCl overnight after cultivation without LiCl to adulthood (third column) are shown. (Fourth column) The individuals shown in the third column were cultivated one more day without LiCl and observed again. For quantification for the experiments shown in the second column, see Supplementary Figure S5D–F. (B–D) Overnight LiCl-induced defects are reversible. Filled circles and filled triangles indicate naïve and continuously LiCl-treated wild-type animals, respectively. Open circles indicate the animals that had been cultivated overnight with LiCl until the first observation on day 1 and thereafter cultivated without LiCl until the second observation on day 2. Statistical analyses were performed on the data for day 2 with Tukey’s (B) or Steel-Dwass (C,D) multiple comparison test. (B) Thermotaxis (n = 3 assays). (C) SNB-1 localization index (n = 10 animals). (D) Fluorescence intensity of GFP∷SYD-2 in the distal region of the neurite. The localization index could not be used in this condition because of the poor S/N ratio due to low fluorescence intensity in LiCl-treated animals (n = 10 animals). (E) Effects of overexpressing ttx-7 on thermotaxis behavior in adult animals after overnight LiCl treatment. Tukey’s multiple comparison test was performed (n ≥ 6 assays). (F) Effects of inositol and other monosaccharides on thermotaxis behavior in adult animals after overnight LiCl treatment. Tukey’s multiple comparison test was performed (n = 4 assays). (G) A model for TTX-7 function. In RIA neurons, the lithium-sensitive enzyme TTX-7/IMPase produces inositol. A supply of inositol is necessary for regulating localization of synaptic components and possibly other cellular functions in mature RIA neurons that together enable the neurons to function as major integrative interneurons for C. elegans behavior.
Similar articles
-
Depressing time: Waiting, melancholia, and the psychoanalytic practice of care.
Salisbury L, Baraitser L. Salisbury L, et al. In: Kirtsoglou E, Simpson B, editors. The Time of Anthropology: Studies of Contemporary Chronopolitics. Abingdon: Routledge; 2020. Chapter 5. In: Kirtsoglou E, Simpson B, editors. The Time of Anthropology: Studies of Contemporary Chronopolitics. Abingdon: Routledge; 2020. Chapter 5. PMID: 36137063 Free Books & Documents. Review.
-
Using Experience Sampling Methodology to Capture Disclosure Opportunities for Autistic Adults.
Love AMA, Edwards C, Cai RY, Gibbs V. Love AMA, et al. Autism Adulthood. 2023 Dec 1;5(4):389-400. doi: 10.1089/aut.2022.0090. Epub 2023 Dec 12. Autism Adulthood. 2023. PMID: 38116059 Free PMC article.
-
Ryan R, Hill S. Ryan R, et al. Cochrane Database Syst Rev. 2019 Oct 23;10(10):ED000141. doi: 10.1002/14651858.ED000141. Cochrane Database Syst Rev. 2019. PMID: 31643081 Free PMC article.
-
Enabling Systemic Identification and Functionality Profiling for Cdc42 Homeostatic Modulators.
Malasala S, Azimian F, Chen YH, Twiss JL, Boykin C, Akhtar SN, Lu Q. Malasala S, et al. bioRxiv [Preprint]. 2024 Jan 8:2024.01.05.574351. doi: 10.1101/2024.01.05.574351. bioRxiv. 2024. PMID: 38260445 Free PMC article. Updated. Preprint.
-
Triana L, Palacios Huatuco RM, Campilgio G, Liscano E. Triana L, et al. Aesthetic Plast Surg. 2024 Oct;48(20):4217-4227. doi: 10.1007/s00266-024-04260-2. Epub 2024 Aug 5. Aesthetic Plast Surg. 2024. PMID: 39103642 Review.
Cited by
-
Goodwin PR, Sasaki JM, Juo P. Goodwin PR, et al. J Neurosci. 2012 Jun 13;32(24):8158-72. doi: 10.1523/JNEUROSCI.0251-12.2012. J Neurosci. 2012. PMID: 22699897 Free PMC article.
-
Neuronal sub-compartmentalization: a strategy to optimize neuronal function.
Donato A, Kagias K, Zhang Y, Hilliard MA. Donato A, et al. Biol Rev Camb Philos Soc. 2019 Jun;94(3):1023-1037. doi: 10.1111/brv.12487. Epub 2019 Jan 4. Biol Rev Camb Philos Soc. 2019. PMID: 30609235 Free PMC article. Review.
-
O'Brien WT, Huang J, Buccafusca R, Garskof J, Valvezan AJ, Berry GT, Klein PS. O'Brien WT, et al. J Clin Invest. 2011 Sep;121(9):3756-62. doi: 10.1172/JCI45194. Epub 2011 Aug 8. J Clin Invest. 2011. PMID: 21821916 Free PMC article.
-
Iwata R, Oda S, Kunitomo H, Iino Y. Iwata R, et al. Proc Natl Acad Sci U S A. 2011 May 3;108(18):7589-94. doi: 10.1073/pnas.1016232108. Epub 2011 Apr 18. Proc Natl Acad Sci U S A. 2011. PMID: 21502506 Free PMC article.
-
Kimata T, Sasakura H, Ohnishi N, Nishio N, Mori I. Kimata T, et al. Worm. 2012 Jan 1;1(1):31-41. doi: 10.4161/worm.19504. Worm. 2012. PMID: 24058821 Free PMC article. Review.
References
-
- Allison, J.H., Stewart, M.A. Reduced brain inositol in lithium-treated rats. Nat. New Biol. 1971;233:267–268. - PubMed
-
- Arimura, N., Kaibuchi, K. Key regulators in neuronal polarity. Neuron. 2005;48:881–884. - PubMed
-
- Atack, J.R., Broughton, H.B., Pollack, S.J. Structure and mechanism of inositol monophosphatase. FEBS Lett. 1995;361:1–7. - PubMed
-
- Bargmann, C.I., Hartwieg, E., Horvitz, H.R. Odorant-selective genes and neurons mediate olfaction in C. elegans. Cell. 1993;74:515–527. - PubMed
-
- Berridge, M.J., Downes, C.P., Hanley, M.R. Neural and developmental actions of lithium: A unifying hypothesis. Cell. 1989;59:411–419. - PubMed
Publication types
MeSH terms
Substances
LinkOut - more resources
Full Text Sources
Molecular Biology Databases
Research Materials