Galphai3 primes the G protein-activated K+ channels for activation by coexpressed Gbetagamma in intact Xenopus oocytes - PubMed
- ️Mon Jan 01 2007
Galphai3 primes the G protein-activated K+ channels for activation by coexpressed Gbetagamma in intact Xenopus oocytes
Moran Rubinstein et al. J Physiol. 2007.
Abstract
G protein-activated K+ channels (GIRK) mediate postsynaptic inhibitory effects of neurotransmitters in the atrium and in the brain by coupling to G protein-coupled receptors (GPCRs). In neurotransmitter-dependent GIRK signalling, Gbetagamma is released from the heterotrimeric Galphabetagamma complex upon GPCR activation, activating the channel and attenuating its rectification. Now it becomes clear that Galpha is more than a mere Gbetagamma donor. We have proposed that Galphai3-GDP regulates GIRK gating, keeping its basal activity low but priming (predisposing) the channel for activation by agonist in intact cells, and by Gbetagamma in excised patches. Here we have further investigated GIRK priming by Galphai3 using a model in which the channel was activated by coexpression of Gbetagamma, and the currents were measured in intact Xenopus oocytes using the two-electrode voltage clamp technique. This method enables the bypass of GPCR activation during examination of the regulation of the channel in intact cells. Using this method, we further characterize the priming phenomenon. We tested and excluded the possibility that our estimates of priming are affected by artifacts caused by series resistance or large K+ fluxes. We demonstrate that both Galphai3 and membrane-attached Gbetagamma scavenger protein, m-phosducin, reduce the basal channel activity. However, Galphai3 allows robust channel activation by coexpressed Gbetagamma, in sharp contrast to m-phosducin, which causes a substantial reduction in the total Gbetagamma-induced current. Furthermore, Galphai3 also does not impair the Gbetagamma-dependent attenuation of the channel rectification, in contrast to m-phosducin, which prevents this Gbetagamma-induced modulation. The Galphai3-induced enhancement of direct activation of GIRK by Gbetagamma, demonstrated here for the first time in intact cells, strongly supports the hypothesis that Galphai regulates GIRK gating under physiological conditions.
Figures
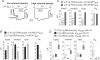
A, representative GIRK currents at a low (left panel) and high expression levels (right panel; note the scale difference). RNAs of GIRK1 and GIRK2 were injected at the same amount, 50 pg RNA per oocyte (left) or 2 ng RNA per oocyte (right). The M2R was expressed at 0.5 ng RNA per oocyte. Solutions were switched as indicated, from physiological solution (ND96, 2 m
mK+) to high K+ (24 m
mK+). Agonist-evoked currents were elicited by perfusing the same solution with the addition of 10 μ
mACh. B, GIRK was injected at low and high densities (50 pg or 2 ng RNA per oocyte). Currents were recorded in different potassium concentrations (24 m
mfor the low density and 6 m
mfor the high density) in order to produce similar inward currents. C, GIRK currents at intermediate density, 0.2 ng RNA per oocyte, in high K+ (24 m
m) or high K+ (96 m
m). D, GIRK was injected at low and high densities (50 pg or 2 ng RNA/oocyte). Currents were recorded with different holding potentials to produce similar inward currents. In B and C, the bars represent means ±
s.e.m.; in D the boxes correspond to the 25–75 percentile range, the middle line is median, and error bars represent the 5–95 percentile range (B-D, n = 6–14). *P < 0.05; and **P < 0.01.
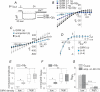
A, the experimental model. Currents from two different oocytes expressing GIRK alone (grey line) or GIRK + Gβγ (black line) are shown. The holding potential was set to −40 mV, and GIRK currents were measured in ND96 and then in the high-K+ (24 m
m)solutions. 5 m
mBa2+ was added at the end of the recording. Breaks are placed where voltage ramps were applied. Under these conditions, basal GIRK activity showed up as an outward current in the 2 m
mK+ solution and as an inward current in 24 m
mK+ (the dotted line shows zero current level). B, I–V curve in high-K+ solution (24 m
m)was obtained using 10 mV voltage steps. GIRK was expressed at low (0.1 ng RNA per oocyte, square) and high densities (2 ng RNA per oocyte, circle) with (dark colour) or without Gβγ (light colour). Gβ was expressed at 5 ng RNA per oocyte and Gγ at 1 ng RNA per oocyte. C, Ba2+ blocks GIRK outward currents less well than inward currents. Currents were recorded in high-K+ solution (24 m
m)supplemented with 5 m
mBa2+ from oocytes expressing GIRK (2 ng RNA per oocyte; line a) or uninjected oocytes (line b). D, I–V curves in high-K+ solution (24 m
m). GIRK was expressed at 2 ng RNA per oocyte. Shown are the total current (c) and net GIRK currents obtained by subtraction of the endogenous currents (averaged from 4 uninjected oocytes, line c-a), or of the currents remaining after the addition of 5 m
mBa2+ (line c-b) n = 4; *P < 0.05. E, normalized GIRK amplitude at −80 mV. F, normalized GIRK slope conductance (GGIRK). G, the comparison of RGβγ, calculated either from currents at −80 mV or from slope conductances, at high and low GIRK densities. RGβγ was calculated in each oocyte using eqn (1) (E) or eqn (2) (F). Note that RGβγ was not normalized. The boxes in E and F correspond to the 25–75 percentile range, the middle line is median, and error bars represent the 5–95 percentile range. The bars in G represent means ±
s.e.m.(E-G, n = 12–14). *P < 0.05; **P < 0.01; and ***P < 0.001.
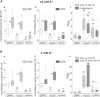
The effects of Gαi3 (1–2 ng RNA per oocyte) or m-phosducin (10 ng RNA per oocyte) on GIRK activation induced by coexpression of Gβγ are summarized from 2–13 oocyte batches (12–85 oocytes). GIRK was expressed at high density (1–2 ng RNA per oocyte). A, summary of measurements in high-K+ solution (24 m
m). Normalized GIRK amplitudes at −80 mV (a) and normalized GGIRK (b) are shown for comparison. Ac, the effects of Gαi3 and m-phosducin on RGβγ. The RGβγ was calculated in each oocyte using eqn (1) or eqn (2). Note that RGβγ was not normalized. B, summary of measurements in low-K+ solution (2 m
m), with normalized amplitude at −60 mV (a) and normalized amplitude at Vrev +50 mV (b). Bc, the effects of Gαi3 and m-phosducin on RGβγ. In all graphs, the boxes correspond to the 25–75 percentile range, the middle line is median, and error bars represent the 5–95 percentile range. All groups were compared both with the control group (GIRK alone; #P < 0.05 and ##P < 0.01) and to all other groups separately (*P < 0.05 and **P < 0.01) using one-way ANOVA, as explained in Methods.
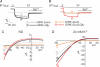
A and B show representative GIRK currents in 4 oocytes of the same batch, expressing GIRK alone (2 ng RNA per oocyte; line 1), GIRK + Gβγ (line 2), GIRK + Gαi3 (line 3) and GIRK + Gαi3 + Gβγ (line 4). The Vhold was −40 mV. The solution changes from ND96 (2 m
mK+) to high K+ (24 m
m)and then to high K+ (24 m
m)with 5 m
mBa2+, as indicated. A voltage ramp between −120 and +50 mV was applied in each solution (indicated by the breaks). C and D show I–V curves obtained by 2 s voltage ramps in ND96 (2 m
mK+; C) or high-K+ solution (24 m
m;D). Net GIRK currents, obtained by subtraction of I–V curves of native (uninjected) oocytes, are shown.
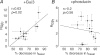
The RGβγ is plotted as a function of percentage decrease in Ibasal recorded in the same batch of oocytes. Each dot represents the mean ±
s.e.m. of percentage decrease in Ibasal and mean ±
s.e.m. RGβγ measured in one oocyte batch (experiment). The percentage decrease in Ibasal was determined in oocytes expressing Gαi3 (A) or m-phosducin (B) relative to oocytes expressing GIRK alone. The RGβγ was determined in oocytes coexpressing Gβγ (Gαi3 + Gβγ or m-phosducin + Gβγ, respectively). A, titrated Gαi3 expression (1 or 2 ng RNA per oocyte) reveals a positive correlation between the percentage decrease in Ibasal and RGβγ (Spearman coefficient 0.63, P = 0.02, 11 oocyte batches). B, titrated m phosducin expression (2–10 ng RNA per oocyte) demonstrates that there is no statistically significant correlation between the percentage decrease in Ibasal and RGβγ (Spearman coefficient −0.2, P = 0.56, 10 oocyte batches). The regression lines are presented for illustration, and do not indicate a linear correlation.
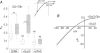
A, the extent of GIRK rectification (expressed at high density, 1–2 ng RNA per oocyte) was measured with the joint expression of Gβγ, Gαi3 or m-phosducin. Currents were recorded in high-K+ solution (24 m
m), the Vrev and amplitudes were determined using 2 s voltage ramp and Fir, the rectification factor, was calculated using eqn (4), as illustrated in the inset. The boxes correspond to the 25–75 percentile range, the middle line is median, and error bars represent the 5–95 percentile range. Data are from 2–9 oocyte batches, 12–68 oocytes. Statistical analysis was performed as explained in the legend to Fig. 4. ## or **, P < 0.01. B, I–V curves, measured in high-K+ solution (24 m
m), in two oocytes expressing different G protein combinations but having similar inward currents (taken from different experiments in which the RNAs of GIRK1 and GIRK2 were injected at 1 ng RNA per oocyte). Despite the similar inward currents, Gαi3 expression reduced the outward current (stronger rectification, grey line) compared to Gαi3 + Gβγ expression (black line). See Supplemental Fig. 3 for a summary of GIRK rectification at similar values of GGIRK.
Similar articles
-
Rubinstein M, Peleg S, Berlin S, Brass D, Keren-Raifman T, Dessauer CW, Ivanina T, Dascal N. Rubinstein M, et al. J Physiol. 2009 Jul 15;587(Pt 14):3473-91. doi: 10.1113/jphysiol.2009.173229. Epub 2009 May 26. J Physiol. 2009. PMID: 19470775 Free PMC article.
-
Gbetagamma-dependent and Gbetagamma-independent basal activity of G protein-activated K+ channels.
Rishal I, Porozov Y, Yakubovich D, Varon D, Dascal N. Rishal I, et al. J Biol Chem. 2005 Apr 29;280(17):16685-94. doi: 10.1074/jbc.M412196200. Epub 2005 Feb 23. J Biol Chem. 2005. PMID: 15728579
-
Kahanovitch U, Tsemakhovich V, Berlin S, Rubinstein M, Styr B, Castel R, Peleg S, Tabak G, Dessauer CW, Ivanina T, Dascal N. Kahanovitch U, et al. J Physiol. 2014 Dec 15;592(24):5373-90. doi: 10.1113/jphysiol.2014.283218. Epub 2014 Nov 10. J Physiol. 2014. PMID: 25384780 Free PMC article.
-
The Roles of Gβγ and Gα in Gating and Regulation of GIRK Channels.
Dascal N, Kahanovitch U. Dascal N, et al. Int Rev Neurobiol. 2015;123:27-85. doi: 10.1016/bs.irn.2015.06.001. Epub 2015 Jul 26. Int Rev Neurobiol. 2015. PMID: 26422982 Review.
-
Molecular mechanisms mediating inhibition of G protein-coupled inwardly-rectifying K+ channels.
Lei Q, Jones MB, Talley EM, Garrison JC, Bayliss DA. Lei Q, et al. Mol Cells. 2003 Feb 28;15(1):1-9. Mol Cells. 2003. PMID: 12661754 Review.
Cited by
-
The role of G proteins in assembly and function of Kir3 inwardly rectifying potassium channels.
Zylbergold P, Ramakrishnan N, Hebert T. Zylbergold P, et al. Channels (Austin). 2010 Sep-Oct;4(5):411-21. doi: 10.4161/chan.4.5.13327. Epub 2010 Sep 1. Channels (Austin). 2010. PMID: 20855978 Free PMC article. Review.
-
Rubinstein M, Peleg S, Berlin S, Brass D, Keren-Raifman T, Dessauer CW, Ivanina T, Dascal N. Rubinstein M, et al. J Physiol. 2009 Jul 15;587(Pt 14):3473-91. doi: 10.1113/jphysiol.2009.173229. Epub 2009 May 26. J Physiol. 2009. PMID: 19470775 Free PMC article.
-
Encephalopathy-causing mutations in Gβ1 (GNB1) alter regulation of neuronal GIRK channels.
Reddy HP, Yakubovich D, Keren-Raifman T, Tabak G, Tsemakhovich VA, Pedersen MH, Shalomov B, Colombo S, Goldstein DB, Javitch JA, Bera AK, Dascal N. Reddy HP, et al. iScience. 2021 Aug 21;24(9):103018. doi: 10.1016/j.isci.2021.103018. eCollection 2021 Sep 24. iScience. 2021. PMID: 34522861 Free PMC article.
-
Inanobe A, Nakagawa A, Kurachi Y. Inanobe A, et al. PLoS One. 2013 Nov 11;8(11):e79844. doi: 10.1371/journal.pone.0079844. eCollection 2013. PLoS One. 2013. PMID: 24244570 Free PMC article.
-
Berlin S, Tsemakhovich VA, Castel R, Ivanina T, Dessauer CW, Keren-Raifman T, Dascal N. Berlin S, et al. J Biol Chem. 2011 Sep 23;286(38):33223-35. doi: 10.1074/jbc.M111.271056. Epub 2011 Jul 27. J Biol Chem. 2011. PMID: 21795707 Free PMC article.
References
-
- Bettahi I, Marker CL, Roman IM, Wickman K. Contribution of the Kir3.1 subunit to the muscarinic-gated atrial potassium channel IKACh. J Biol Chem. 2002;277:48282–48288. - PubMed
-
- Bichet D, Cornet V, Geib S, Carlier E, Volsen S, Hoshi T, Mori Y, De Waard M. The I–II loop of the Ca2+ channel α1 subunit contains an endoplasmic reticulum retention signal antagonized by the β subunit. Neuron. 2000;25:177–190. - PubMed
Publication types
MeSH terms
Substances
LinkOut - more resources
Full Text Sources