MicroRNA-mediated regulation of stomatal development in Arabidopsis - PubMed
MicroRNA-mediated regulation of stomatal development in Arabidopsis
Claudia Kutter et al. Plant Cell. 2007 Aug.
Erratum in
- Plant Cell. 2007 Nov;19(11):3831
Abstract
The proper number and distribution of stomata are essential for the efficient exchange of gases between the atmosphere and the aerial parts of plants. We show that the density and development of stomatal complexes on the epidermis of Arabidopsis thaliana leaves depend, in part, on the microRNA-mediated regulation of Agamous-like16 (AGL16), which is a member of the MADS box protein family. AGL16 mRNA is targeted for sequence-specific degradation by miR824, a recently evolved microRNA conserved in the Brassicaceae and encoded at a single genetic locus. Primary stomatal complexes can give rise to higher-order complexes derived from satellite meristemoids. Expression of a miR824-resistant AGL16 mRNA, but not the wild-type AGL16 mRNA, in transgenic plants increased the incidence of stomata in higher-order complexes. By contrast, reduced expression of AGL16 mRNA in the agl16-1 deficiency mutant and in transgenic lines overexpressing miR824 decreased the incidence of stomata in higher-order complexes. These findings and the nonoverlapping patterns of AGL16 mRNA and miR824 localization led us to propose that the miR824/AGL16 pathway functions in the satellite meristemoid lineage of stomatal development.
Figures
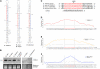
Fold Back Structure, Expression, and Conservation of miR824 in the Brassicaceae. (A) 5′ to 3′ stem-loop hairpin structures of the pre-miR824 predicted for Arabidopsis and three closely related Brassica species. The mature miR824 sequence is indicated in red, and the miR824* sequence is indicated in blue. G:U wobble pairing is shown by a circle. Note that the miR824/miR824* sequences are identical in Arabidopsis and the Brassica species. (B) RNA blot hybridization of miR824 in leaves of Brassica species, Arabidopsis Col-0, O. sativa, and N. benthamiana. The 5S rRNA and tRNA loading standards are stained with ethidium bromide. The size of the RNA is indicated at left in nucleotides (nt). (C) RNA ligase–mediated rapid amplification of cDNA ends (RLM-RACE) mapping of AGL16 mRNA cleavage sites in Col-0. The arrow indicates the site of cleavage in 10 of 10 sequenced clones. Perfect base pairing between miR824 and AGL16 mRNA is shown by vertical lines; G:U wobble pairing is indicated by a circle. The predicted free energy of pairing between miR824 and AGL16 mRNA is −38.6 kcal/mol. Conserved miRNA binding sites (red), conserved nucleotides (black), and nonconserved nucleotides (gray highlight) in Arabidopsis and Brassica species are indicated. (D) to (F) Sequence complementarity between transcripts encoded by miRNA genes and their targets. The red line in (D) indicates complementarity between MIR824 and AGL16 in Arabidopsis. The orange line in (E) indicates complementarity between the MIR824 ortholog in B. oleracea (MIRBr824) and its target sequence AGL16. The blue lines in (F) indicate complementarity between MIR171 and its two targets genes, SCL6-III and SCL6-IV, in Arabidopsis. The gray line in each panel indicates the expected background complementarity at each position in the duplex (see Supplemental Methods online). The percentage of paired bases in a window of 20 nucleotides is shown for a potential duplex structure between miRNA precursor and target sequences. The duplex was anchored at the miRNA complementary site, indicated by vertical dashed lines. Values for each window are plotted aligned to its center position. Sequences of miRNA gene transcripts and their target RNAs, 88 nucleotides in length and centered on the miRNA complementary site, are shown below each plot with the miRNAs highlighted in red.
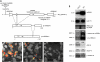
Expression of the MIR824 Locus in the Wild Type and in the Overexpression Mutant m3. (A) Schematic representation of the MIR824 locus (At4g24415.1) on chromosome IV based on the alignment of ESTs with genomic sequences (see Supplemental Figure 2A online). Exons (open boxes), introns (horizontal lines), start of transcription (+1), transcript length, and the position and orientation of miR824 (short arrows) are indicated. The scheme is not drawn to scale. (B) to (D) Transient expression experiments with pProMIR824:Luc constructs. Col-0 plants were bombarded with pPro2x35S:Luc carrying a luciferase gene regulated by the Pro2x35S promoter (B), pProMIR824:Luc carrying 2954 bp of genomic region upstream of the pri-miR824 start of transcription fused to the luciferase gene of pProMIR824:Luc (C), and pLuc carrying a promoterless luciferase/35S terminator cassette (D). Yellow to red false color indicates increasing levels of luciferase activity detected at 48 h after bombardment. (E) RNA gel blot hybridization of miR824 in 20 μg of low molecular weight RNA and AGL16 mRNA in 2 μg of poly(A)+ RNA prepared from leaves of Col-0 and m3. tRNA and 5S rRNA were stained with ethidium bromide. The fold expression of RNA in m3 relative to Col-0 normalized for β-TUBULIN mRNA is indicated. The sizes of the RNAs are shown at left in nucleotides (nt).
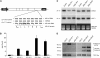
Expression of miR824 and AGL16 mRNA in AGL16.1/2 and AGL16m1/2 Leaves. (A) Nucleotide sequence of AGL16 (AGL16m) with silent mutations in the miR824 recognition site. miR824 pairs to AGL16 RNA at the nucleotide segment corresponding to amino acids Ser-197 and Leu-203. Predicted free energies of pairing to miR824 are indicated at left. Mutated nucleotides are underlined. Vertical lines indicate perfect base pairing, circles indicate G:U wobble pairing, and gray shading indicates the HincII restriction site (GTT/GAC) introduced into AGL16m. (B) Fold expression relative to the wild type of uncleaved target AGL16 mRNA (black bars) and SCL6-III mRNA (gray bars), determined by RT–quantitative PCR using primers spanning the miR824 and the miR171 complementary sites, was normalized to TIP41-like (At4g34270) mRNA. (C) RNA gel blot hybridization of low molecular weight RNA in leaves of Col-0, AGL16.1/2, and AGL16m1/2 using probes for miR824 and miR171. tRNA and 5S rRNA were stained with ethidium bromide. The sizes of the RNAs are indicated at left in nucleotides (nt). (D) AGL16 and AGL16m transcripts were distinguished by HincII digestion, which only digests AGL16m cDNA, after RT-PCR amplification of endogenous and AGL16m transcripts.
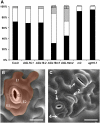
Effects of Altered AGL16 Expression on the Proportion of Primary and Higher-Order Stomatal Complexes. (A) Relative proportion of primary (black bars), secondary (white bars), tertiary (gray bars), and quaternary (striped bars) stomatal complexes on the abaxial surfaces of the fifth rosette leaves of Col-0, AGL16.1/2, AGL16m1/2, m3, and agl16-1 plants. At least 110 stomatal complexes were scored for each line. Asterisks above the bars indicate distributions significantly different (P < 5 × 10−5) from the Col-0 distribution by the χ2 test. (B) and (C) Representative scanning electron microscopy images of stomatal complexes on the abaxial surfaces of fifth rosette leaves. Bars = 10 μm. (B) A primary Col-0 stomatal complex consisting of a central pair of guard cells (G1 and G2) and stoma surround by neighboring cells (E1, E2, and E3). (C) A quaternary stomatal complex of AGL16m1 with primary, secondary, tertiary, and quaternary complexes. The numbered arrows indicate the apparent order in which stomata form. Note that the fourth order stomata is at the early, satellite meristemoid stage of development.
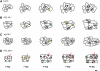
Time Course of Stomatal Development on the Abaxial Surfaces of First Rosette Leaves. Drawings of the relevant features of nail polish pictures taken from sequential dental resin impressions showing the development of representative stomatal complexes on the abaxial surfaces of first true leaves of Col-0 (A), AGL16.1 (B), agl16-1 (C), and AGL16m1 (D) plants monitored daily starting at 6 d after germination (dpg). Satellite meristemoids are colored in green, guard mother cells in yellow, kidney-shaped guard cells in gray, and jigsaw-shaped epidermal cells are not colored. Bar = 10 μm.
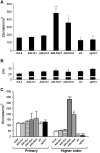
Effects of Altered AGL16 mRNA Expression on Stomatal Density and Stomatal Index. (A) and (B) Average stomatal density (A) and average stomatal index (B) (SI%) ±
semfor three to four replicates of the abaxial surfaces of fifth rosette leaves. (C) Average densities of primary stomatal complexes and higher-order stomatal complexes calculated from the individual measurements in (A) and the proportion of stomatal types in Figure 4A. Significance levels (t test of means) relative to the Col-0 controls were as follows: * P < 0.05, ** P < 0.025, *** P < 0.01, and **** P < 0.005.
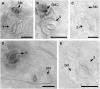
Expression Patterns of miR824 and AGL16 mRNA in Stomatal Complexes. In situ hybridization of the abaxial epidermis of first true leaves of B. rapa imaged at 1 week after germination. Differential interference contrast images show whole mounts hybridized with probes for sense miR824 ([A] and [B]), antisense miR824 (C), sense AGL16 mRNA (D), and antisense AGL16 mRNA (E). Regions hybridizing with the probe appear dark gray. Mature stomata (S), satellite meristemoids (SM), and guard mother cell (GMC) are indicated. Bars = 10 μm.
Similar articles
-
Yang K, Jiang M, Le J. Yang K, et al. J Integr Plant Biol. 2014 Jun;56(6):539-49. doi: 10.1111/jipb.12154. Epub 2014 Mar 6. J Integr Plant Biol. 2014. PMID: 24386951
-
Szaker HM, Darkó É, Medzihradszky A, Janda T, Liu HC, Charng YY, Csorba T. Szaker HM, et al. Front Plant Sci. 2019 Nov 25;10:1454. doi: 10.3389/fpls.2019.01454. eCollection 2019. Front Plant Sci. 2019. PMID: 31824525 Free PMC article.
-
Zhao PX, Miao ZQ, Zhang J, Chen SY, Liu QQ, Xiang CB. Zhao PX, et al. J Exp Bot. 2020 Oct 7;71(19):6092-6106. doi: 10.1093/jxb/eraa303. J Exp Bot. 2020. PMID: 32594177
-
Take a deep breath: peptide signalling in stomatal patterning and differentiation.
Richardson LG, Torii KU. Richardson LG, et al. J Exp Bot. 2013 Dec;64(17):5243-51. doi: 10.1093/jxb/ert246. Epub 2013 Aug 30. J Exp Bot. 2013. PMID: 23997204 Review.
-
Plant development: YODA the stomatal switch.
Gray JE, Hetherington AM. Gray JE, et al. Curr Biol. 2004 Jun 22;14(12):R488-90. doi: 10.1016/j.cub.2004.06.019. Curr Biol. 2004. PMID: 15203025 Review.
Cited by
-
Zluhan-Martínez E, Pérez-Koldenkova V, Ponce-Castañeda MV, Sánchez MP, García-Ponce B, Miguel-Hernández S, Álvarez-Buylla ER, Garay-Arroyo A. Zluhan-Martínez E, et al. Int J Mol Sci. 2020 Jul 12;21(14):4925. doi: 10.3390/ijms21144925. Int J Mol Sci. 2020. PMID: 32664691 Free PMC article. Review.
-
Stomatal development and movement: the roles of MAPK signaling.
Liu YK, Liu YB, Zhang MY, Li DQ. Liu YK, et al. Plant Signal Behav. 2010 Oct;5(10):1176-80. doi: 10.4161/psb.5.10.12757. Epub 2010 Oct 1. Plant Signal Behav. 2010. PMID: 20855958 Free PMC article. Review.
-
mmannot: How to improve small-RNA annotation?
Zytnicki M, Gaspin C. Zytnicki M, et al. PLoS One. 2020 May 28;15(5):e0231738. doi: 10.1371/journal.pone.0231738. eCollection 2020. PLoS One. 2020. PMID: 32463818 Free PMC article.
-
SPEECHLESS Speaks Loudly in Stomatal Development.
Chen L, Wu Z, Hou S. Chen L, et al. Front Plant Sci. 2020 Feb 21;11:114. doi: 10.3389/fpls.2020.00114. eCollection 2020. Front Plant Sci. 2020. PMID: 32153616 Free PMC article. Review.
-
The interaction between OsMADS57 and OsTB1 modulates rice tillering via DWARF14.
Guo S, Xu Y, Liu H, Mao Z, Zhang C, Ma Y, Zhang Q, Meng Z, Chong K. Guo S, et al. Nat Commun. 2013;4:1566. doi: 10.1038/ncomms2542. Nat Commun. 2013. PMID: 23463009 Free PMC article.
References
-
- Allen, E., Xie, Z., Gustafson, A.M., Sung, G.H., Spatafora, J.W., and Carrington, J.C. (2004). Evolution of microRNA genes by inverted duplication of target gene sequences in Arabidopsis thaliana. Nat. Genet. 36 1282–1290. - PubMed
-
- Alvarez-Buylla, E.R., Liljegren, S.J., Pelaz, S., Gold, S.E., Burgeff, C., Ditta, G.S., Vergara-Silva, F., and Yanofsky, M.F. (2000). MADS-box gene evolution beyond flowers: Expression in pollen, endosperm, guard cells, roots, and trichomes. Plant J. 24 457–466. - PubMed
Publication types
MeSH terms
Substances
LinkOut - more resources
Full Text Sources
Other Literature Sources
Molecular Biology Databases