Regulation of the Xenopus Xsox17alpha(1) promoter by co-operating VegT and Sox17 sites - PubMed
- ️Mon Jan 01 2007
Regulation of the Xenopus Xsox17alpha(1) promoter by co-operating VegT and Sox17 sites
Laura Howard et al. Dev Biol. 2007.
Abstract
The gene encoding the Sox F-group transcription factor Xsox17alpha(1) is specifically expressed throughout the entire region of the Xenopus blastula fated to become endoderm, and is important in controlling endodermal development. Xsox17alpha(1) is a direct target of the maternal endodermal determinant VegT and of Sox17 itself. We have analysed the promoter of the Xenopus laevis Xsox17alpha(1) gene by transgenesis, and have identified two important control elements which reside about 9 kb upstream at the start of transcription. These elements individually drive transgenic endodermal expression in the blastula and gastrula. One contains functional, cooperating VegT and Sox-binding consensus sites. The Sox sites in this region are occupied in vivo. The other responds to TGF-beta signals like Activin or Nodals that act through Smad2/3. We propose that these two regions co-operate in regulating the early endodermal expression of the Xsox17alpha(1) gene.
Figures
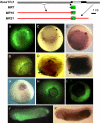
Initial transgenics made with large fragments of the Xsox17α1 promoter. Top: Map of the endogenous gene, with its single intron, and below are maps of two GFP constructs with large fragments of the Xsox17α1 promoter (red), and also a small promoter fragment with just 260 bp of upstream sequence (black). (A, B) Fluorescent expression of the MR21 construct in stage 10.5 gastrulae. (C) In situ hybridisation to GFP mRNA from MR19 in an embryo similar to that in panels A, and (D) an optical section of this embryo after clearing. (E, F) In situ to the endogenous Xsox17 transcripts for comparison. The blastopore lip is marked by an arrowhead in panels A–F. At stage 12, the endogenous gene is expressed as in panel G, and the MR21 GFP is shown in panel H. A minimal promoter, with only 260 bp of 5′ sequence is not expressed in the vegetal region (I), but it is expressed elsewhere. (J) MR21 is expressed throughout the endoderm at tailbud stages, just like the endogenous gene, as shown in an in situ hybridisation (K). The arrowheads mark the blastopore.
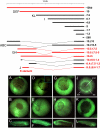
Transgenic expression of deletion constructs. Top: Diagrams of a series of 5′ and internal deletion fragments fused to GFP, as in Fig. 1. The endodermal element is marked and fragments giving vegetal expression in the early gastrula are colored red. Below is GFP expression in various transgenics, with the corresponding expression panels indicated on the left of the constructs. (A, D, G, J, K) are stage 10.5; (B, E, H) are stage 12; (C, F, I, L) are tailbud stages. (A–C) Construct − 12Δ10-5 is negative in early endoderm. (D–F) Positive construct − 10; the embryo in (D) is a hemi-transgenic, providing a good control for the background fluorescence; the insert panel is the animal pole at the same exposure. (G–H) Positive construct − 10.5Δ7.7-5. (I) Construct − 5.7 is positive in the later foregut, as well as in the axis, like other 5′ constructs; (J) − 9.5Δ8.4-1.7 is positive in the involuting and non-involuting endoderm, whereas − 7.5 is not (K), but it gives low-level expression in the posterior endoderm of the tailbud (L). In panel J, the main tissues of the early gastrula are marked: end, endoderm; ect, ectoderm; ee, the extra-blastoporal endoderm, which involutes over the blastopore.
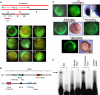
(A) Dissection of the E-element. At the top are the sub-regions of the E-element that were tested in GFP transgenics, attached to a cytoskeletal-type muscle actin basal promoter. All constructs except C3 overlapped to avoid disrupting a possible control element. Images of the transgenics are shown below, labelled by construct, the basal promoter alone being labelled BAct (Latinkic et al., 2002). Expression of the entire E-element is shown in Fig. 2J. (B, C) Mutational analysis of the B1 element. In panel B are maps of selected transcription factor binding sites in B1, and also C3. The sequences of B1 and C3 are shown in Supplementary Fig. 3. In panel C is a transgenic analysis of mutants of B1 in which the transcription factor binding sites were disrupted. The green panels show GFP fluorescence and the others show in situ hybridisation to GFP mRNA. The third B1 panel shows a cleared embryo, with GFP expression in the deep involuting endoderm and also the extra-blastoporal, epithelial, involuting endoderm, enlarged in the fourth panel. The arrow indicates the blastopore lip. (D) Electrophoretic mobility shift analysis of B1 T-box motif. EMSA assays were conducted with the variant VegT-binding sequence in B1, with a mutant of it and with the consensus sequence in the Derrière gene. In each case, the reactions were performed with or without competitor (±).
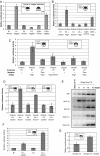
Transient transgenic analysis of the B1 and C3 promoter elements using luciferase constructs. In all experiments, the vegetal samples injected with DNA constructs or the animal poles injected with DNA plus mRNA are normalised relative to control animal hemisphere expression of the same DNA construct alone (set to 1.0). Each individual test plasmid firefly luciferase measurement is first normalised with respect to an internal Renilla luciferase control. (A) Animal versus vegetal expression of the endodermal elements. (B) Response of endodermal elements to VegT in animal hemispheres. (C) Effect of mutating the T-box site on B1 response to VegT. The first four tracks show expression in the embryonic animal hemisphere, the last three show expression in the oocyte to measure direct effects of VegT (see text). Co-injection into the embryo of mRNAs encoding VegT and dominant negative Actin receptor (tXAR) demonstrates reduced induction by VegT when TGF-β signalling is blocked. (D) Responses of B1 and C3 to Xsox17 mRNA. (E) RT–PCR showing time course of gene induction by Xsox17 mRNA in animal caps. Expression in control animal caps is the same at all stages. (F) Activin responsiveness of B1 and C3 in animal caps. The constructs, with and without 20 pg Activin mRNA were injected into animal poles and the embryos analysed at stage 10.5. (G) C3 was injected into the oocyte nucleus together with 200 pg activated Smad2 mRNA.
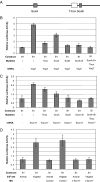
Mutational and anti-sense analysis of B1. (A) Position of the T-box and Sox response elements in B1. (B) Responses of B1 mutants to VegT mRNA, assayed at stage 10.5. All DNAs, together with the appropriate mRNAs, were injected into animal hemispheres and the luciferase activity normalised to each DNA injected alone (set to 1.0). Only the unmutated B1 without VegT bar is shown, since all controls were set to 1.0. (C) Response of B1 and its mutants to Xsox17α1, assayed at stage 17. (D) The stimulation of B1 in the vegetal compared to the animal pole is eliminated by injecting the anti-sense morpholino oligos against α1, α2 and β Xsox17 mRNAs.
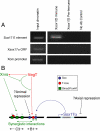
(A) Chromatin immunoprecipitation of the B1 element. Chromatin from stage 11 gastrulae was precipitated with an anti-Xsox17β antiserum, the DNA extracted and subjected to PCR using primers to the B1 plus C3 elements, the Xsox17α ORF and the Xom promoter, which should not be regulated by Xsox17. (B) Summary of the regulation of the Xsox17α1 promoter. VegT induces Xsox17α directly, via the T-box half site in B1, as well as nodal-related proteins (red). However, the Xsox17 induction becomes inhibited by a VegT-derived inhibitor. The inhibition is over-ridden by TGFβ signals, induced by VegT. This may simply be by the positive induction of C3 via FoxH1/Smad sites (green). Xsox17 autoregulates itself synergistically through Sox binding sites (blue) and the adjacent T-box half site (red). In addition, these Sox sites might respond to repressive members of the Sox family, restricting expression to the vegetal pole.
Similar articles
-
SOX7 is an immediate-early target of VegT and regulates Nodal-related gene expression in Xenopus.
Zhang C, Basta T, Fawcett SR, Klymkowsky MW. Zhang C, et al. Dev Biol. 2005 Feb 15;278(2):526-41. doi: 10.1016/j.ydbio.2004.11.008. Dev Biol. 2005. PMID: 15680368
-
Ahmed N, Howard L, Woodland HR. Ahmed N, et al. Differentiation. 2004 Apr;72(4):171-84. doi: 10.1111/j.1432-0436.2004.07204005.x. Differentiation. 2004. PMID: 15157240
-
Yasuo H, Lemaire P. Yasuo H, et al. Curr Biol. 1999 Aug 26;9(16):869-79. doi: 10.1016/s0960-9822(99)80391-1. Curr Biol. 1999. PMID: 10469589
-
Charney RM, Paraiso KD, Blitz IL, Cho KWY. Charney RM, et al. Semin Cell Dev Biol. 2017 Jun;66:12-24. doi: 10.1016/j.semcdb.2017.03.003. Epub 2017 Mar 22. Semin Cell Dev Biol. 2017. PMID: 28341363 Free PMC article. Review.
-
Walentek P. Walentek P. Genesis. 2021 Feb;59(1-2):e23406. doi: 10.1002/dvg.23406. Epub 2021 Jan 5. Genesis. 2021. PMID: 33400364 Review.
Cited by
-
Trinh LT, Osipovich AB, Sampson L, Wong J, Wright CVE, Magnuson MA. Trinh LT, et al. iScience. 2022 Aug 11;25(9):104905. doi: 10.1016/j.isci.2022.104905. eCollection 2022 Sep 16. iScience. 2022. PMID: 36046192 Free PMC article.
-
Control of zygotic genome activation in Xenopus.
Blitz IL, Cho KWY. Blitz IL, et al. Curr Top Dev Biol. 2021;145:167-204. doi: 10.1016/bs.ctdb.2021.03.003. Epub 2021 Apr 19. Curr Top Dev Biol. 2021. PMID: 34074529 Free PMC article. Review.
-
Uncovering the mesendoderm gene regulatory network through multi-omic data integration.
Jansen C, Paraiso KD, Zhou JJ, Blitz IL, Fish MB, Charney RM, Cho JS, Yasuoka Y, Sudou N, Bright AR, Wlizla M, Veenstra GJC, Taira M, Zorn AM, Mortazavi A, Cho KWY. Jansen C, et al. Cell Rep. 2022 Feb 15;38(7):110364. doi: 10.1016/j.celrep.2022.110364. Cell Rep. 2022. PMID: 35172134 Free PMC article.
-
Mukherjee S, Chaturvedi P, Rankin SA, Fish MB, Wlizla M, Paraiso KD, MacDonald M, Chen X, Weirauch MT, Blitz IL, Cho KW, Zorn AM. Mukherjee S, et al. Elife. 2020 Sep 7;9:e58029. doi: 10.7554/eLife.58029. Elife. 2020. PMID: 32894225 Free PMC article.
-
Favarolo MB, Revinski DR, Garavaglia MJ, López SL. Favarolo MB, et al. Life Sci Alliance. 2022 Sep 30;5(12):e202201693. doi: 10.26508/lsa.202201693. Life Sci Alliance. 2022. PMID: 36180230 Free PMC article.
References
-
- Afouda B.A., Ciau-Uitz A., Patient R. GATA4, 5 and 6 mediate TGF{beta} maintenance of endodermal gene expression in Xenopus embryos. Development. 2005;132:763–774. - PubMed
-
- Ahmed N., Howard L., Woodland H.R. Early endodermal expression of the Xenopus Endodermin gene is driven by regulatory sequences containing essential Sox protein-binding elements. Differentiation. 2004;72:171–184. - PubMed
-
- Aoki T.O., David N.B., Minchiotti G., Saint-Etienne L., Dickmeis T., Persico G.M., Strahle U., Mourrain P., Rosa F.M. Molecular integration of casanova in the Nodal signalling pathway controlling endoderm formation. Development. 2002;129:275–286. - PubMed
-
- Chen X., Weisberg E., Fridmacher V., Watanabe M., Naco G., Whitman M. Smad4 and FAST-1 in the assembly of activin-responsive factor. Nature. 1997;389:85–89. - PubMed
-
- Clements D., Woodland H.R. Changes in embryonic cell fate produced by expression of an endodermal transcription factor, Xsox17. Mech. Dev. 2000;99:65–70. - PubMed
Publication types
MeSH terms
Substances
LinkOut - more resources
Full Text Sources
Molecular Biology Databases
Miscellaneous