Characterization of a human coagulation factor Xa-binding site on Viperidae snake venom phospholipases A2 by affinity binding studies and molecular bioinformatics - PubMed
- ️Mon Jan 01 2007
Characterization of a human coagulation factor Xa-binding site on Viperidae snake venom phospholipases A2 by affinity binding studies and molecular bioinformatics
Grazyna Faure et al. BMC Struct Biol. 2007.
Abstract
Background: The snake venom group IIA secreted phospholipases A2 (SVPLA2), present in the Viperidae snake family exhibit a wide range of toxic and pharmacological effects. They exert their different functions by catalyzing the hydrolysis of phospholipids (PL) at the membrane/water interface and by highly specific direct binding to: (i) presynaptic membrane-bound or intracellular receptors; (ii) natural PLA2-inhibitors from snake serum; and (iii) coagulation factors present in human blood.
Results: Using surface plasmon resonance (SPR) protein-protein interaction measurements and an in vitro biological test of inhibition of prothrombinase activity, we identify a number of Viperidae venom SVPLA2s that inhibit blood coagulation through direct binding to human blood coagulation factor Xa (FXa) via a non-catalytic, PL-independent mechanism. We classify the SVPLA2s in four groups, depending on the strength of their binding. Molecular electrostatic potentials calculated at the surface of 3D homology-modeling models show a correlation with inhibition of prothrombinase activity. In addition, molecular docking simulations between SVPLA2 and FXa guided by the experimental data identify the potential FXa binding site on the SVPLA2s. This site is composed of the following regions: helices A and B, the Ca2+ loop, the helix C-beta-wing loop, and the C-terminal fragment. Some of the SVPLA2 binding site residues belong also to the interfacial binding site (IBS). The interface in FXa involves both, the light and heavy chains.
Conclusion: We have experimentally identified several strong FXa-binding SVPLA2s that disrupt the function of the coagulation cascade by interacting with FXa by the non-catalytic PL-independent mechanism. By theoretical methods we mapped the interaction sites on both, the SVPLA2s and FXa. Our findings may lead to the design of novel, non-competitive FXa inhibitors.
Figures
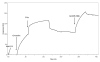
The interaction between an isoform of CTX and FXa, as measured by SPR. The anti-CA monoclonal antibody mAb A-73.13 [70] was covalently attached to the chip [71]. CTX (the CA2-CBc complex) was then captured via this mAb before injection of FXa (25 μg/ml). FXa bound to CTX, as seen in the rise of the resonance signal, showing that the CTX-FXa complex remained attached to the anti-CA mAb. After injection of a specific anti-CB MAb (B-32.13, 10 μg/ml), the signal increased further, confirming the presence of CB in the ternary CA-CB-FXa complex.
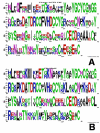
Weblogo representation of the multiple sequence alignment of the SVPLA2s. A. Group NB (AGTX, Catx and CbI). Each logo consists of stacks of symbols, one stack for each position in the sequence. The overall height of the stack indicates the sequence conservation at that position, while the height of symbols within the stack indicates the relative frequency of each amino acid at that position. The symbols in each stack are arranged by alphabetical order from top to bottom and do not follow the order in which the sequences were fed. Arg, His and Lys residues are in blue; Asp and Glu in red; Ala, Ile, Leu, Met, Phe, Pro, Trp, and Val in black; Gly, Cys, Ser, Thr, and Tyr, in green; Asn, Gln in purple. Renetseder numbering system for all PLA2s throughout this work [15]. B. Group VS (CBc and MtxII).
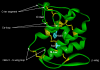
Ribbon diagram of the molecular model of AtxA. α-helices A, B, C and D, and the β-wing are labeled. The seven-disulfide bridges are in yellow sticks and the His48/Asp99 pair in cyan sticks.
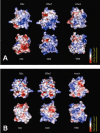
MEP at the solvent accessible surface of the 3D molecular models of the Viperidae SVPLA2s. A. The models correspond to CBc, CBa2, AtxA, CbI, CbII, and Vbb. Color codes correspond to MEP in kT/e units: blue, +5; red, -5; white, 0. Front view. B. Back view.
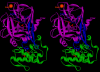
Ribbon representation of the 3D molecular model of the complex between AtxA and the light and heavy chains of FXa. Crossed-eye stereo ribbon representation, with AtxA in green, the light and heavy chains of FXa in blue and purple, respectively. Catalytic site residues are red sticks for both AtxA (His48, Asp99) and FXa (His57, Ser102, Asp195). The metal Ca2+ ion of FXa is depicted as an orange sphere. The N- and C-ter of the AtxA, FXa's heavy chain and FXa's light chain are indicated.
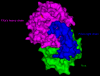
Solvent-accessible surface representation of the 3D molecular model of the complex between AtxA and the light and heavy chains of FXa. Color coding as in Fig. 5.
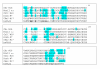
Viperidae SVPLA2 interface amino acid residues. Interface amino acid residues of the SVPLA2 complexes with FXa (CBc, MtxII, CbII, AtxA and CBa2). Underlined characters denote residues identified by mutagenesis to be critical for binding to FXa and inhibition of prothrombinase activity [39, 51]. Bold characters denote residues defining the IBS of hsPLA2 [13, 14]. Cyan-shadowed characters denote residues found at the interface of the selected complexes. The alignment reflects Renetseder's numbering system.
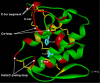
Ribbon diagram of the molecular model of AtxA showing the identified interface regions. The identified interface regions in SVPLA2s from Fig. 7 and made up of consensus positions 2, 3, 7 (helix A); 16; 18, 19 (helix B); 23, 24; 31–34 (Ca2+ loop); 53, 59, 60, 69, 70 (helix C-β-wing loop); and 118, 119, 121–124, 129–131, 133 (C-terminal segment), are in red (see Results section).
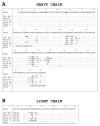
FXa interface amino acid residues. A. Amino acid residues of the heavy chain of FXa at the interface of the selected docked complexes for each SVPLA2. The sequence of FXa shows only residues detected in the X-ray experiment. The last line shows the experimentally reported residues of FXa involved in the binding to FVa [2, 78, 79, 83]. We use Renetseder's notation for the SVPLA2s and chymotrypsinogen notation for FXa. The sequences of CBc, AtxA, CbII and CBa2 were aligned with respect to MtxII. B. Amino acid residues of the light chain of FXa at the interface of the selected docked complexes for each SVPLA2.
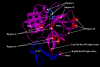
Ribbon diagram of the crystal structure of FXa (PDB 2BOH) showing the identified interface regions. The figure highlights in red the regions corresponding to the identified interface residues from Fig. 9A and B. In the light chain: Arg86-Ser90 and Cys100-Asn105. In the heavy chain: Region I: Arg93; Phe101 (from the 99-loop). Region II: Arg125; Asp126; Glu129-Ser130; Thr134. Region III: Tyr162; Asp164-Asn166; Lys169; Leu170. Region IV: Gln178 and Asn179 (from the 174-loop). Region V: Lys230, Thr232, Ala233, Phe234 and Lys236 (residues from the C-terminal helix). Only residues present three or more times in the same column in the sequences are included. Light chain is in blue, heavy chain in purple. FXa is rotated 90° in a clockwise sense about its vertical axes with respect to Fig. 5. The terminal ends of FXa's chains are labeled. The catalytic triad is represented as cyan sticks.
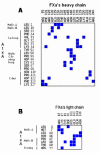
Contact map for the AtxA-FXa complex. A. Heavy-chain FXa residues. Only residues for which the contact area is equal or greater than 10 Å2 are shown.B. Light-chain FXa residues.
Similar articles
-
Saul FA, Prijatelj-Znidarsic P, Vulliez-le Normand B, Villette B, Raynal B, Pungercar J, Krizaj I, Faure G. Saul FA, et al. J Struct Biol. 2010 Mar;169(3):360-9. doi: 10.1016/j.jsb.2009.10.010. Epub 2009 Oct 24. J Struct Biol. 2010. PMID: 19857576
-
Mounier CM, Luchetta P, Lecut C, Koduri RS, Faure G, Lambeau G, Valentin E, Singer A, Ghomashchi F, Béguin S, Gelb MH, Bon C. Mounier CM, et al. Eur J Biochem. 2000 Aug;267(16):4960-9. doi: 10.1046/j.1432-1327.2000.01523.x. Eur J Biochem. 2000. PMID: 10931177
-
Kini RM. Kini RM. Toxicon. 2005 Jun 15;45(8):1147-61. doi: 10.1016/j.toxicon.2005.02.018. Epub 2005 Apr 13. Toxicon. 2005. PMID: 15922780 Review.
-
Faure G, Saul F. Faure G, et al. Acta Chim Slov. 2011 Dec;58(4):671-7. Acta Chim Slov. 2011. PMID: 24061114 Review.
Cited by
-
Animal Toxins: A Historical Outlook at the Institut Pasteur of Paris.
Popoff MR, Faure G, Legout S, Ladant D. Popoff MR, et al. Toxins (Basel). 2023 Jul 19;15(7):462. doi: 10.3390/toxins15070462. Toxins (Basel). 2023. PMID: 37505731 Free PMC article. Review.
-
Secreted Phospholipases A2 - not just Enzymes: Revisited.
Ivanušec A, Šribar J, Križaj I. Ivanušec A, et al. Int J Biol Sci. 2022 Jan 1;18(2):873-888. doi: 10.7150/ijbs.68093. eCollection 2022. Int J Biol Sci. 2022. PMID: 35002531 Free PMC article. Review.
-
Nemecz D, Ostrowski M, Ravatin M, Saul F, Faure G. Nemecz D, et al. Molecules. 2020 Nov 13;25(22):5290. doi: 10.3390/molecules25225290. Molecules. 2020. PMID: 33202772 Free PMC article.
-
Xie C, Slagboom J, Albulescu LO, Bruyneel B, Still KBM, Vonk FJ, Somsen GW, Casewell NR, Kool J. Xie C, et al. Toxins (Basel). 2020 Jan 16;12(1):53. doi: 10.3390/toxins12010053. Toxins (Basel). 2020. PMID: 31963329 Free PMC article.
-
Isolated biomolecules of pharmacological interest in hemostasis from Cerastes cerastes venom.
Chérifi F, Laraba-Djebari F. Chérifi F, et al. J Venom Anim Toxins Incl Trop Dis. 2013 May 1;19(1):11. doi: 10.1186/1678-9199-19-11. J Venom Anim Toxins Incl Trop Dis. 2013. PMID: 23849477 Free PMC article.
References
-
- Davie EW. Biochemical and molecular aspects of the coagulation cascade. Thromb Haemost. 1995;74:1–6. - PubMed
-
- Sabharwal AK, Padmanabhan K, Tulinsky A, Mathur A, Gorka J, Bajaj SP. Interaction of calcium with native and decarboxylated human factor X. Effect of proteolysis in the autolysis loop on catalytic efficiency and factor Va binding. J Biol Chem. 1997;272:22037–22045. doi: 10.1074/jbc.272.35.22037. - DOI - PubMed
MeSH terms
Substances
LinkOut - more resources
Full Text Sources
Other Literature Sources
Miscellaneous