Dynamic remodeling of individual nucleosomes across a eukaryotic genome in response to transcriptional perturbation - PubMed
- ️Tue Jan 01 2008
Dynamic remodeling of individual nucleosomes across a eukaryotic genome in response to transcriptional perturbation
Sushma Shivaswamy et al. PLoS Biol. 2008.
Abstract
The eukaryotic genome is packaged as chromatin with nucleosomes comprising its basic structural unit, but the detailed structure of chromatin and its dynamic remodeling in terms of individual nucleosome positions has not been completely defined experimentally for any genome. We used ultra-high-throughput sequencing to map the remodeling of individual nucleosomes throughout the yeast genome before and after a physiological perturbation that causes genome-wide transcriptional changes. Nearly 80% of the genome is covered by positioned nucleosomes occurring in a limited number of stereotypical patterns in relation to transcribed regions and transcription factor binding sites. Chromatin remodeling in response to physiological perturbation was typically associated with the eviction, appearance, or repositioning of one or two nucleosomes in the promoter, rather than broader region-wide changes. Dynamic nucleosome remodeling tends to increase the accessibility of binding sites for transcription factors that mediate transcriptional changes. However, specific nucleosomal rearrangements were also evident at promoters even when there was no apparent transcriptional change, indicating that there is no simple, globally applicable relationship between chromatin remodeling and transcriptional activity. Our study provides a detailed, high-resolution, dynamic map of single-nucleosome remodeling across the yeast genome and its relation to global transcriptional changes.
Conflict of interest statement
Competing interests. The authors have declared that no competing interests exist.
Figures
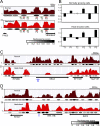
(A) Detailed view of the PHO5 locus showing the raw sequence reads (brown and red profiles). The nucleosome positions calculated using our analysis algorithm are shown as ovals, shaded according to their nucleosome score as indicated. The positions of the amplicons used for qPCR analysis are marked as red (peaks) and green (troughs) lines below. The black arrows indicate the positions of genes in that region. (B) qPCR verification of the three nucleosome peaks and three troughs identified by sequencing confirm that their positions remain the same before and after heat shock. (C) The heat-shock–induced SSA4 gene and flanking regions, showing that nucleosomes are displaced specifically at the SSA4 promoter and coding region after heat shock (thick purple arrow). (D) The heat-shock–repressed ribosomal protein gene RPL17B and flanking regions, showing that a single positioned nucleosome appears after heat shock specifically at the RPL17B promoter (thin purple arrow). The nucleosome positions calculated using our analysis algorithm are indicated as in (A).
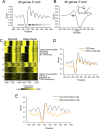
(A) Average nucleosome profiles of all genes in the yeast genome from −600 bp to +1,000 bp with respect to the transcription start site (TSS). Nucleosome positions are shown as gray ovals below the profile. The intensity of the filled oval reflects the average probability score of the nucleosomes (see Figure 1 for the color scale), and the dotted oval marks the spread of that nucleosome across all genes. (B) The 3′ end of genes is marked by a strongly positioned nucleosome, followed by a relatively nucleosome-free region. The inset shows the 3′ end of convergently transcribed genes in which the 3′ end is not followed by another promoter. (C) Distinct classes of nucleosome profiles revealed by k-means clustering of all promoters in the yeast genome. Each row in the clusters shows the position of a nucleosome at an individual promoter. Nucleosomes are colored according to their probability using the shown color scale. Clusters 1–3 showed a significant enrichment for genes with lower transcription rates and for TATA-less genes (p ≤ 10−10). Cluster 6 showed a significant enrichment for genes with high transcription rates and for TATA-containing genes (p < 10−10). (D) Average nucleosome profiles for TATA-containing (973) and TATA-less (4,382) promoters, aligned with respect to the TSS. (E) The genes in the yeast genome were sorted in descending order according to their transcription rates [16], and the average promoter nucleosome profiles for the top 500 genes (orange) and the bottom 500 genes (black) are plotted.
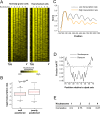
(A) Genes were aligned to the first nucleosome downstream of the TSS and sorted by their nucleosome positioning periodicity (NPP) score (see Materials and Methods). Genes were sorted by their NPP scores in normally growing cells, and the data from heat-shocked cells are shown in the same order. The unaligned TSS is indicated by the approximate curve. (B) The transcription rate of genes with high NPP scores (well-positioned nucleosomes) is significantly lower than that of genes with low NPP scores (poorly positioned). In these box plots, the red line indicates the median, the upper and lower bounds of the box indicate the interquartile range, the horizontal lines that are connected to the box by a dashed line indicate the upper and lower bounds of nonoutlier values, and the open circles indicate outliers. (C) Genes were sorted in descending order according to their transcription rates, and the average nucleosome profiles over the coding regions for top 500 genes (orange) and the bottom 500 genes (black) are plotted. (D) Frequency of AA/TT dinucleotide at each position in the DNA sequence associated with the most strongly positioned first nucleosomes. The frequency profiles for the dinucleotides AA and TT for the first nucleosome shown in (A) were summed and smoothed using a 3-bp moving average. The same analysis was also performed for a comparable set of randomly chosen DNA sequences from the yeast genome. (E) Correlation coefficients of the AA/TT profiles for the DNA sequences underlying each of the indicated coding nucleosome positions from (A), with the positioning profile derived earlier. Each of the correlation values was significantly higher than background.
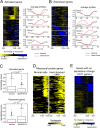
All profiles are aligned with respect to the TSS. (A) Remodeling profiles of genes activated greater than 2-fold after heat shock and (B), genes repressed greater than 2-fold by heat shock. Nucleosomes present during normal growth but evicted by heat shock are indicated in yellow, and nucleosomes that appeared after heat shock are shown in blue. The average profiles of nucleosomes in each group before and after heat shock are shown on the right. The k-means clustering for (A) and (B) was done based on data from −200 to TSS, but data are shown for −300 to +100. (C) A remodeling score for eviction and for appearance was separately calculated for activated genes and repressed genes (Materials and Methods), and the data were plotted using box plots similar to Figure 3B. Activated genes showed significantly higher eviction scores than appearance scores, whereas repressed genes showed significantly higher appearance scores than eviction scores. (D) Nucleosome positions at the promoters of ribosomal protein genes during normal growth and after heat shock, clustered on data from −200 to +100 bp. (E) Remodeling profiles of genes whose expression changed by less than 1.2-fold after heat shock, clustered based on data from −300 to +100 bp.
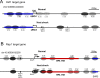
(A) Example of nucleosome eviction at the heat-shock–activated UBC4 promoter (blue line). Nucleosomes defined by our sequencing data are indicated by ovals, colored according to their stability score. The positions of transcription factor binding sites are from [17] and are shaded according to their confidence. Binding sites for other transcription factors are also affected by remodeling (unpublished data), but these are not known to be related to heat shock. (B) Example of nucleosome appearance at the heat-shock–repressed RPL17B promoter (red line).
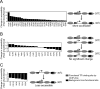
Transcription factors were classified into three groups based on the change in accessibility of their functional binding sites because of nucleosome repositioning after heat shock. Graphs of accessibility changes in arbitrary units (see Materials and Methods) are plotted for transcription factor binding sites that (A) showed an increase in accessibility, (B) showed no significant change in accessibility, and (C) showed a decrease in accessibility upon heat shock. The right of each graph shows a schematic of the relationship between nucleosomes and transcription factor binding sites.
Similar articles
-
Zawadzki KA, Morozov AV, Broach JR. Zawadzki KA, et al. Mol Biol Cell. 2009 Aug;20(15):3503-13. doi: 10.1091/mbc.e09-02-0111. Epub 2009 Jun 3. Mol Biol Cell. 2009. PMID: 19494041 Free PMC article.
-
Wu X, Liu H, Liu H, Su J, Lv J, Cui Y, Wang F, Zhang Y. Wu X, et al. Gene. 2013 Nov 1;530(1):8-18. doi: 10.1016/j.gene.2013.08.018. Epub 2013 Aug 16. Gene. 2013. PMID: 23958656
-
Weakly positioned nucleosomes enhance the transcriptional competency of chromatin.
Belch Y, Yang J, Liu Y, Malkaram SA, Liu R, Riethoven JJ, Ladunga I. Belch Y, et al. PLoS One. 2010 Sep 24;5(9):e12984. doi: 10.1371/journal.pone.0012984. PLoS One. 2010. PMID: 20886052 Free PMC article.
-
Nucleosome positioning in yeasts: methods, maps, and mechanisms.
Lieleg C, Krietenstein N, Walker M, Korber P. Lieleg C, et al. Chromosoma. 2015 Jun;124(2):131-51. doi: 10.1007/s00412-014-0501-x. Epub 2014 Dec 23. Chromosoma. 2015. PMID: 25529773 Review.
-
Barrera LO, Ren B. Barrera LO, et al. Curr Opin Cell Biol. 2006 Jun;18(3):291-8. doi: 10.1016/j.ceb.2006.04.002. Epub 2006 May 2. Curr Opin Cell Biol. 2006. PMID: 16647254 Review.
Cited by
-
Hughes AL, Jin Y, Rando OJ, Struhl K. Hughes AL, et al. Mol Cell. 2012 Oct 12;48(1):5-15. doi: 10.1016/j.molcel.2012.07.003. Epub 2012 Aug 9. Mol Cell. 2012. PMID: 22885008 Free PMC article.
-
Genomic Nucleosome Organization Reconstituted with Pure Proteins.
Krietenstein N, Wal M, Watanabe S, Park B, Peterson CL, Pugh BF, Korber P. Krietenstein N, et al. Cell. 2016 Oct 20;167(3):709-721.e12. doi: 10.1016/j.cell.2016.09.045. Cell. 2016. PMID: 27768892 Free PMC article.
-
Chang GS, Noegel AA, Mavrich TN, Müller R, Tomsho L, Ward E, Felder M, Jiang C, Eichinger L, Glöckner G, Schuster SC, Pugh BF. Chang GS, et al. Genome Res. 2012 Jun;22(6):1098-106. doi: 10.1101/gr.131649.111. Epub 2012 Mar 20. Genome Res. 2012. PMID: 22434426 Free PMC article.
-
An integrated machine-learning model to predict nucleosome architecture.
Sala A, Labrador M, Buitrago D, De Jorge P, Battistini F, Heath IB, Orozco M. Sala A, et al. Nucleic Acids Res. 2024 Sep 23;52(17):10132-10143. doi: 10.1093/nar/gkae689. Nucleic Acids Res. 2024. PMID: 39162225 Free PMC article.
-
Shan X, Roberts C, Lan Y, Percec I. Shan X, et al. Sci Rep. 2018 Jul 31;8(1):11502. doi: 10.1038/s41598-018-29775-y. Sci Rep. 2018. PMID: 30065345 Free PMC article.
References
-
- Luger K, Mader AW, Richmond RK, Sargent DF, Richmond TJ. Crystal structure of the nucleosome core particle at 2.8 A resolution. Nature. 1997;389:251–260. - PubMed
-
- Yuan GC, Liu YJ, Dion MF, Slack MD, Wu LF, et al. Genome-scale identification of nucleosome positions in S. cerevisiae. Science. 2005;309:626–630. - PubMed
Publication types
MeSH terms
Substances
LinkOut - more resources
Full Text Sources
Other Literature Sources
Molecular Biology Databases