DNA damage induced by chronic inflammation contributes to colon carcinogenesis in mice - PubMed
. 2008 Jul;118(7):2516-25.
doi: 10.1172/JCI35073.
James M Bugni, Stephanie L Green, Chung-Wei Lee, Bo Pang, Diana Borenshtein, Barry H Rickman, Arlin B Rogers, Catherine A Moroski-Erkul, Jose L McFaline, David B Schauer, Peter C Dedon, James G Fox, Leona D Samson
Affiliations
- PMID: 18521188
- PMCID: PMC2423313
- DOI: 10.1172/JCI35073
DNA damage induced by chronic inflammation contributes to colon carcinogenesis in mice
Lisiane B Meira et al. J Clin Invest. 2008 Jul.
Abstract
Chronic inflammation increases cancer risk. While it is clear that cell signaling elicited by inflammatory cytokines promotes tumor development, the impact of DNA damage production resulting from inflammation-associated reactive oxygen and nitrogen species (RONS) on tumor development has not been directly tested. RONS induce DNA damage that can be recognized by alkyladenine DNA glycosylase (Aag) to initiate base excision repair. Using a mouse model of episodic inflammatory bowel disease by repeated administration of dextran sulfate sodium in the drinking water, we show that Aag-mediated DNA repair prevents colonic epithelial damage and reduces the severity of dextran sulfate sodium-induced colon tumorigenesis. Importantly, DNA base lesions expected to be induced by RONS and recognized by Aag accumulated to higher levels in Aag-deficient animals following stimulation of colonic inflammation. Finally, as a test of the generality of this effect we show that Aag-deficient animals display more severe gastric lesions that are precursors of gastric cancer after chronic infection with Helicobacter pylori. These data demonstrate that the repair of DNA lesions formed by RONS during chronic inflammation is important for protection against colon carcinogenesis.
Figures
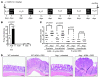
(A) Treatment scheme. White blocks represent 16 days of normal water except the last which is 7 days. Black blocks represent 5 days of 2.5% DSS in water except for the last, which was 4 days of 2% DSS. Mice were also treated with only AOM or only DSS. (B) Tumor multiplicity in Aag+/+ animals (white), and Aag–/– animals (black). AOM+DSS Aag+/+, n = 12; AOM+DSS Aag–/–, n = 23; AOM Aag+/+, n = 15; AOM Aag–/–, n = 19; DSS Aag+/+, n = 10; and DSS Aag–/–, n = 10. Data are mean ± SD. (C) Pathology scores for Aag+/+ (circles) and Aag–/– (diamonds) mice. See Supplemental Methods for a complete description of scoring criteria. Lines indicate the median. (D) Photomicrographs depicting, from left to right, normal untreated Aag+/+ colon; AOM+DSS-treated Aag+/+ colon bearing a lesion with an average dysplasia score, a sessile adenoma; normal untreated Aag–/– colon; and AOM+DSS-treated Aag–/– colon bearing a lesion with an average dysplasia score and a higher scoring pedunculated adenoma. Scale bars: 200 μm.
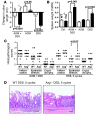
(A) Change in colon length. (B) Spleen weight as a percentage of body weight. AOM+DSS Aag+/+, n = 14; AOM+DSS Aag–/–, n = 31; AOM Aag+/+, n = 15; AOM Aag–/–, n = 19; DSS Aag+/+, n = 10; DSS Aag–/–, n = 10. Data are mean ± SD. (C) Histopathology scores for severity of inflammation, epithelial defects, and crypt atrophy. A description of the histopathological endpoints for inflammation examined can be found in Supplemental Methods. Epithelial defect scores were based on increased gland dilation and surface epithelial attenuation. (D) Photomicrographs showing a DSS-treated Aag+/+ colon bearing a lesion with an average epithelial defect score (left) and a DSS-treated Aag–/– colon bearing a lesion with an average epithelial defect score (right). Scale bars: 100 μm.
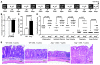
(A) Treatment scheme. (B) Decrease in colon length for Aag–/– animals (n = 18) compared with Aag+/+ animals (n = 7). (C) Increase in spleen weight as a percentage of body weight for Aag+/+ (n = 7) and Aag–/– animals (n = 18). Data are mean ± SD. (D) Pathology scores for Aag+/+ (circles) and Aag–/– (diamonds) mice. (E) Histopathology of colonic disease induced by 7 cycles of DSS. From left to right: Aag+/+ colon exhibited moderate inflammation and glandular epithelial hyperplasia; Aag+/+ colon exhibited mild dysplasia characterized by epithelial cell pleomorphism and mild branching, with hyperplasia and inflammation; Aag–/– colon exhibited moderate to severe inflammation, crypt atrophy, mucosal collapse, and segmental epithelial cell loss; Aag–/– colon exhibited a portion of an intraepithelial neoplasia with mucosal dysplasia characterized by loss of columnar orientation, elongation, branching and infolding, glandular ectasia, inflammation, and crypt abscesses. Scale bars: 100 μm.
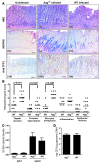
(A) Histopathology of gastric disease and mucosal metaplasia induced by H. pylori infection in the stomach mucosa of Aag+/+ or Aag–/– mice. Uninfected Aag+/+ mouse stomach showed normal microscopic architecture (H&E) and a thin surface lining of gastric-type neutral mucins (red; AB/PAS), and anti-TFF2 stained intermittent mucous neck cells within normal oxyntic mucosa. Uninfected Aag–/– mice were indistinguishable from the uninfected Aag+/+, so only the Aag+/+ is shown. For infected Aag–/– mice, moderate gastritis with marked mucous metaplasia, hyperplasia, and oxyntic atrophy (loss of parietal and chief cells) was observed (H&E). Hyperplastic mucous neck cell population replaced resident zymogenic cells, secreting a mixture of gastric (red) and intestinal-type (acidic, blue) mucins (AB/PAS). Mucous metaplasia associated with expanded mucous neck cell population highlighted by TFF2 immunoreactivity. For infected Aag+/+ mice, gastritis but minimal oxyntic alterations were observed (H&E). Mucous neck cells were significantly reduced in number in the Aag+/+ H. pylori–infected mouse (AB/PAS). Fewer TFF2-positive mucous neck cells were observed in the infected Aag+/+ mouse. Scale bars: 160 μm. (B) Pathology scores for infected Aag+/+ (circles) and infected Aag–/– (diamonds) mice. (C) Serum IgG1 and IgG2c responses to H. pylori in Aag–/– and Aag+/+ mice 32 weeks after infection. (D) H. pylori colonization levels in the stomach. Data presented as mean ± SD of log-transformed CFU/μg of genomic DNA.
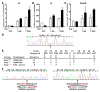
Six- to 8-week-old male mice were untreated or were administered 2.5% DSS for 5 days and euthanized 1 or 7 days after treatment. Levels of the modified bases εA (A), εC (B), and 8oxoG (C) were measured from colonic mucosal DNA and are shown for Aag-proficient (+) and Aag-deficient (–) mice. Within treatment groups, we observed no differences between Aag+/+ and Aag–/– mice, and thus these genotypes were combined in the analysis. Data are mean ± SD. (D–F) Point mutations in Ctnnb1. (D and E) Chromatogram showing an example of the most common mutation observed, a G:C to A:T transition in codon 41 of Ctnnb1. The arrow indicates the peaks for the mutant and WT sequences. (E and F) Distribution of mutations in Ctnnb1. Base substitutions are indicated below the sequence, and their frequencies are shown for the different experimental groups. (F) Deletion mutants in Ctnnb1. Chromatograms of the 2 deletion mutants are shown with an arrow below the sequence indicating the deletion junction. Also shown is the deleted sequence (lowercase) with red highlighting of regions of microhomology between the deleted portion and the adjacent retained sequence.
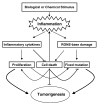
Inflammatory cells produce cytokines that induce proliferation and suppress apoptosis. The toxic effects of RONS can partially counterbalance these signals, leading to cell death or an inability to repopulate eroded regions. Either cytotoxicity or cytostasis would require neighboring cells to replicate for healing to occur, and under chronic conditions, cell death could further stimulate inflammation. In addition, survival factors act in concert with RONS-induced miscoding or replication blocking DNA lesions to fix and expand cancer-causing mutations.
Similar articles
-
Aag-initiated base excision repair promotes ischemia reperfusion injury in liver, brain, and kidney.
Ebrahimkhani MR, Daneshmand A, Mazumder A, Allocca M, Calvo JA, Abolhassani N, Jhun I, Muthupalani S, Ayata C, Samson LD. Ebrahimkhani MR, et al. Proc Natl Acad Sci U S A. 2014 Nov 11;111(45):E4878-86. doi: 10.1073/pnas.1413582111. Epub 2014 Oct 27. Proc Natl Acad Sci U S A. 2014. PMID: 25349415 Free PMC article.
-
DNA repair is indispensable for survival after acute inflammation.
Calvo JA, Meira LB, Lee CY, Moroski-Erkul CA, Abolhassani N, Taghizadeh K, Eichinger LW, Muthupalani S, Nordstrand LM, Klungland A, Samson LD. Calvo JA, et al. J Clin Invest. 2012 Jul;122(7):2680-9. doi: 10.1172/JCI63338. Epub 2012 Jun 11. J Clin Invest. 2012. PMID: 22684101 Free PMC article.
-
Sayed IM, Sahan AZ, Venkova T, Chakraborty A, Mukhopadhyay D, Bimczok D, Beswick EJ, Reyes VE, Pinchuk I, Sahoo D, Ghosh P, Hazra TK, Das S. Sayed IM, et al. J Biol Chem. 2020 Aug 7;295(32):11082-11098. doi: 10.1074/jbc.RA119.009981. Epub 2020 Jun 9. J Biol Chem. 2020. PMID: 32518160 Free PMC article.
-
Helicobacter pylori-Mediated Genetic Instability and Gastric Carcinogenesis.
Shimizu T, Chiba T, Marusawa H. Shimizu T, et al. Curr Top Microbiol Immunol. 2017;400:305-323. doi: 10.1007/978-3-319-50520-6_13. Curr Top Microbiol Immunol. 2017. PMID: 28124159 Review.
-
Molecular Mechanisms of H. pylori-Induced DNA Double-Strand Breaks.
Kidane D. Kidane D. Int J Mol Sci. 2018 Sep 23;19(10):2891. doi: 10.3390/ijms19102891. Int J Mol Sci. 2018. PMID: 30249046 Free PMC article. Review.
Cited by
-
Application of liposomes in drug development--focus on gastroenterological targets.
Zhang JX, Wang K, Mao ZF, Fan X, Jiang DL, Chen M, Cui L, Sun K, Dang SC. Zhang JX, et al. Int J Nanomedicine. 2013;8:1325-34. doi: 10.2147/IJN.S42153. Epub 2013 Apr 8. Int J Nanomedicine. 2013. PMID: 23630417 Free PMC article. Review.
-
Millar-Wilson A, Ward Ó, Duffy E, Hardiman G. Millar-Wilson A, et al. iScience. 2022 Oct 26;25(11):105421. doi: 10.1016/j.isci.2022.105421. eCollection 2022 Nov 18. iScience. 2022. PMID: 36388986 Free PMC article. Review.
-
Adenosine, bridging chronic inflammation and tumor growth.
Chen L, Alabdullah M, Mahnke K. Chen L, et al. Front Immunol. 2023 Oct 31;14:1258637. doi: 10.3389/fimmu.2023.1258637. eCollection 2023. Front Immunol. 2023. PMID: 38022572 Free PMC article. Review.
-
Mangerich A, Knutson CG, Parry NM, Muthupalani S, Ye W, Prestwich E, Cui L, McFaline JL, Mobley M, Ge Z, Taghizadeh K, Wishnok JS, Wogan GN, Fox JG, Tannenbaum SR, Dedon PC. Mangerich A, et al. Proc Natl Acad Sci U S A. 2012 Jul 3;109(27):E1820-9. doi: 10.1073/pnas.1207829109. Epub 2012 Jun 11. Proc Natl Acad Sci U S A. 2012. PMID: 22689960 Free PMC article.
-
Gobert AP, Verriere T, de Sablet T, Peek RM Jr, Chaturvedi R, Wilson KT. Gobert AP, et al. Cell Microbiol. 2013 Jan;15(1):145-56. doi: 10.1111/cmi.12039. Epub 2012 Nov 7. Cell Microbiol. 2013. PMID: 23051580 Free PMC article.
References
-
- Parkin D.M., Bray F., Ferlay J., Pisani P. Global cancer statistics, 2002. CA Cancer J. Clin. 2005;55:74–108. - PubMed
Publication types
MeSH terms
Substances
Grants and funding
LinkOut - more resources
Full Text Sources
Other Literature Sources
Molecular Biology Databases