Alternative pathways for production of beta-amyloid peptides of Alzheimer's disease - PubMed
Review
Alternative pathways for production of beta-amyloid peptides of Alzheimer's disease
Vivian Hook et al. Biol Chem. 2008 Aug.
Abstract
This highlight article describes three Alzheimer's disease (AD) studies presented at the 5th General Meeting of the International Proteolysis Society that address enzymatic mechanisms for producing neurotoxic beta-amyloid (Abeta) peptides. One group described the poor kinetics of BACE 1 for cleaving the wild-type (WT) beta-secretase site of APP found in most AD patients. They showed that cathepsin D displays BACE 1-like specificity and cathepsin D is 280-fold more abundant in human brain than BACE 1. Nevertheless, as BACE 1 and cathepsin D show poor activity towards the WT beta-secretase site, they suggested continuing the search for additional beta-secretase(s). The second group reported cathepsin B as an alternative beta-secretase possessing excellent kinetic efficiency and specificity for the WT beta-secretase site. Significantly, inhibitors of cathepsin B improved memory, with reduced amyloid plaques and decreased Abeta(40/42) in brains of AD animal models expressing amyloid precursor protein containing the WT beta-secretase site. The third group addressed isoaspartate and pyroglutamate (pGlu) posttranslational modifications of Abeta. Results showed that cathepsin B, but not BACE 1, efficiently cleaves the WT beta-secretase isoaspartate site. Furthermore, cyclization of N-terminal Glu by glutaminyl cyclase generates highly amyloidogenic pGluAbeta(3-40/42). These presentations suggest cathepsin B and glutaminyl cyclase as potential new AD therapeutic targets.
Figures
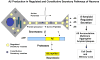
Aβ peptides are generated by proteolytic processing of the amyloid precursor protein (APP) in secretory vesicles that undergo axonal transport from the neuronal cell body to nerve terminals, where Aβ is secreted. Extracellular Aβ peptides accumulate as oligomers and aggregates in amyloid plaques, and cause loss of memory in Alzheimer’s disease. Cleavage at the N-terminus of Aβ within APP is achieved by proteases known as β-secretases, and cleavage at the C-termini of Aβ within APP is achieved by γ-secretases. Proteolytic processing by β- and γ-secretases results in Aβ peptides of 40 and 42 residues, known as Aβ(1-40) and Aβ(1-42). In addition, APP can be modified to contain isoaspartate (isoAsp) residues, which generates Aβ containing isoAsp, isoAβ(40/42). Moreover, Aβ can be truncated and the terminal glutamate residue modified to pyroglutamate (pGlu) to form pGluAβ(3-40/42). The β-secretases that produce these various forms of Aβ and the enzyme which generates pGlu, are the subject of this report.
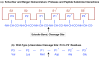
. The active site of the enzyme is composed of several subsites. The scheme shows an active site of six subsites, termed S1 to S3 and S1’ to S3’. Subsites are located on both sides of the catalytic site and are numbered from this point in either direction. The positions of amino acid residues of the hexapeptide substrate are counted from the point of cleavage and thus have the same numbering as the subsites they occupy (P1 to P3 and P1’ to P3’). Cleavage always occurs between P1 and P1’ (Schechter and Berger, 1967).
b. P3 to P3’ residues at the wild-type β-secretase cleavage site. Cleavage of the wild-type site β-secretase site of APP occurs between Met-↓Asp which represent the P1-P1’ residues. The Val-Lys-Met residues represent the P3, P2, and P1 residues, respectively; the Asp-Ala-Glu residues are the P1’, P2’, and P3’ residues, respectively.
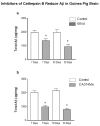
E64d or CA074Me were administered by constant icv infusion into guinea pig brains by ALZET minipumps for 7 days or 30 days. Brain extracts were prepared and total Aβ (Aβ40 and Aβ42) were measured by ELISA assays.
(a) E64d reduces brain Aβ levels. E64d treatment for 7 or 30 days reduced the total Aβ by 31% and 55% relative to control, respectively (diagonal striped bars). Results are shown as mean ± SEM (pg/mg protein), with * indicating a significant difference from controls (p < 0.05, student’s t-test).
(b) CA074Me reduces brain Aβ levels. In vivo CA074Me treatment for 7 or 30 days reduced total Aβ by 57% and 62% relative to control, respectively (solid bars). After 7 days of treatment, CA074Me significantly reduced total Aβ. Data are shown as mean ± SEM (pg/mg protein), with statistical significance with * indicating a significant difference from controls (p < 0.05, student’s t-test).
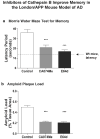
. The CA074Me and E64d inhibitors of cathepsin B were administered (icv) to the London APP mice that express human APP with the wild-type β-secretase site and a mutation near the γ-secretase site. The constant icv administration into the brain utilized Alzet minipumps for 28 days. Mice were then evaluated for the status of memory by the Morris water maze test; the latency period measures the time required for the animal to swim to a submerged platform after training to learn of the platform location, with shorter times reflecting improved memory. The mean latency times and percent standard errors (% SEM) are shown for the control (vehicle treated), CA074Me-treated, and E64d-treated London APP animals. The statistical significance between the means for the London APP animals of each treated group and the control is indicated (*** p < 0.0001, student’s t-test). The mean latency period for untreated wild-type, strain-matched, non-transgenic mice is shown as a dashed line. Thus, the CA074Me and E64d treatments significantly shortened the latency period by 42% and 53%, respectively, relative to the London APP controls and restored about 68% and 86%, respectively, of the memory of wild-type non-transgenic mice.
b. Inhibitors of cathepsin B reduce amyloid plaque load in the London APP mice. Reduction of total amyloid plaque load occured in brains after treatment of London APP mice with CA074Me or E64d inhibitors. After treatment with these inhibitors (as described in previous paragraph), amyloid plaques in brain were revealed by anti-Aβ immunohistochemistry of brain sections. Quantitation of relative amyloid plaque load in the entire brain section was achieved by computer image analyses, expressed as percent of the tissue area. The relative amyloid loads are displayed as the mean (% tissue area) and SEM. Statistical significance between each inhibitor-treated group and control is indicated (*** p < 0.0001, student’s t-test). Inhibitor treatments reduce the plaque load by about 45%.
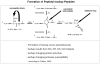
The spontaneous occurring chemical rearrangement of asparagine or aspartate residues depends on the structure of the adjacent C-terminal amino acid. It proceeds very fast if Asp is followed by small aliphatic amino acids such as Gly, Ser or Ala (half-times of minutes). The majority of the isoAsp-residues have been found on the protein surface, since the more rigid protein core prevents this intramolecular reaction. The enzyme Peptidyl-isoAspartyl Methyltransferase can convert only within a peptide chain isoAsp back into Asp (Clarke, S., 2003).
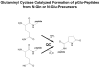
The N-terminal glutamate residue of Aβ(3-40/42) peptide undergoes modification to pyroglutamate (pGlu) by glutaminyl cyclase to generate pGluAβ(3-40/42) peptide forms of Aβ. Glutaminyl cyclase catalyzes the formation of pGlu-peptides from N-Glu- or N-Gln-peptide precursors. The resultant modified pGluAβ(3-40/42) peptides represent a significant portion of Aβ peptides of amyloid plaques in Alzheimer’s disease brains (Roher et al., 1993; Saido et al., 1995; Saido et al., 1996; Schilling et al., 2004).
Similar articles
-
Böhme L, Hoffmann T, Manhart S, Wolf R, Demuth HU. Böhme L, et al. Biol Chem. 2008 Aug;389(8):1055-66. doi: 10.1515/BC.2008.125. Biol Chem. 2008. PMID: 18979630
-
Gandhi S, Refolo LM, Sambamurti K. Gandhi S, et al. J Mol Neurosci. 2004;24(1):137-43. doi: 10.1385/JMN:24:1:137. J Mol Neurosci. 2004. PMID: 15314262
-
Hook G, Yu J, Toneff T, Kindy M, Hook V. Hook G, et al. J Alzheimers Dis. 2014;41(1):129-49. doi: 10.3233/JAD-131370. J Alzheimers Dis. 2014. PMID: 24595198 Free PMC article.
-
Hook V, Hook G, Kindy M. Hook V, et al. Biol Chem. 2010 Aug;391(8):861-72. doi: 10.1515/BC.2010.110. Biol Chem. 2010. PMID: 20536395 Free PMC article. Review.
-
De-Paula VJ, Radanovic M, Diniz BS, Forlenza OV. De-Paula VJ, et al. Subcell Biochem. 2012;65:329-52. doi: 10.1007/978-94-007-5416-4_14. Subcell Biochem. 2012. PMID: 23225010 Review.
Cited by
-
Chia Seed Does Not Improve Cognitive Impairment in SAMP8 Mice Fed with High Fat Diet.
Rui Y, Lv M, Chang J, Xu J, Qin L, Wan Z. Rui Y, et al. Nutrients. 2018 Aug 14;10(8):1084. doi: 10.3390/nu10081084. Nutrients. 2018. PMID: 30110883 Free PMC article.
-
Schwenck J, Maurer A, Fehrenbacher B, Mehling R, Knopf P, Mucha N, Haupt D, Fuchs K, Griessinger CM, Bukala D, Holstein J, Schaller M, Menendez IG, Ghoreschi K, Quintanilla-Martinez L, Gütschow M, Laufer S, Reinheckel T, Röcken M, Kalbacher H, Pichler BJ, Kneilling M. Schwenck J, et al. Theranostics. 2019 May 31;9(13):3903-3917. doi: 10.7150/thno.31037. eCollection 2019. Theranostics. 2019. PMID: 31281521 Free PMC article.
-
Vitória JJM, Trigo D, da Cruz E Silva OAB. Vitória JJM, et al. Cell Mol Life Sci. 2022 Jan 28;79(2):101. doi: 10.1007/s00018-021-04090-4. Cell Mol Life Sci. 2022. PMID: 35089425 Free PMC article. Review.
-
Functional amyloids as natural storage of peptide hormones in pituitary secretory granules.
Maji SK, Perrin MH, Sawaya MR, Jessberger S, Vadodaria K, Rissman RA, Singru PS, Nilsson KP, Simon R, Schubert D, Eisenberg D, Rivier J, Sawchenko P, Vale W, Riek R. Maji SK, et al. Science. 2009 Jul 17;325(5938):328-32. doi: 10.1126/science.1173155. Epub 2009 Jun 18. Science. 2009. PMID: 19541956 Free PMC article.
-
Hook G, Jacobsen JS, Grabstein K, Kindy M, Hook V. Hook G, et al. Front Neurol. 2015 Sep 2;6:178. doi: 10.3389/fneur.2015.00178. eCollection 2015. Front Neurol. 2015. PMID: 26388830 Free PMC article. Review.
References
-
- Beck M, Bigl V, Rossner S. Guinea pigs as a nontransgenic model for APP processing in vitro and in vivo. Neurochem Res. 2003;28:637–644. - PubMed
-
- Beck M, Muller D, Bigl V. Amyloid precursor protein in guinea pigs-complete cDNA sequence and alternative splicing. Biochim Biophys Acta. 1997;1351:17–21. - PubMed
-
- Benjannet S, Savaria D, Laslop A, Munzer JS, Chretien M, Marcinkiewicz M, Seidah NG. Alpha1-antitrypsin Portland inhibits processing of precursors mediated by proprotein convertases primarily within the constitutive secretory pathway. J Biol Chem. 1997;272:26210–26218. - PubMed
-
- Berg JM, Tymoczko JL, Stryer L. Biochemistry. W.H Freeman and Company; New York: 2002. pp. 203–204.
-
- Blennow K, de Leon MJ, Zetterberg H. Alzheimer’s disease. Lancet. 2006;368:387–403. - PubMed
Publication types
MeSH terms
Substances
Grants and funding
LinkOut - more resources
Full Text Sources
Other Literature Sources
Medical