Application of a translational profiling approach for the comparative analysis of CNS cell types - PubMed
- ️Tue Jan 01 2008
. 2008 Nov 14;135(4):749-62.
doi: 10.1016/j.cell.2008.10.029.
Joseph D Dougherty, Myriam Heiman, Eric F Schmidt, Tanya R Stevens, Guojun Ma, Sujata Bupp, Prerana Shrestha, Rajiv D Shah, Martin L Doughty, Shiaoching Gong, Paul Greengard, Nathaniel Heintz
Affiliations
- PMID: 19013282
- PMCID: PMC2763427
- DOI: 10.1016/j.cell.2008.10.029
Application of a translational profiling approach for the comparative analysis of CNS cell types
Joseph P Doyle et al. Cell. 2008.
Erratum in
- Cell. 2009 Nov 25;139(5):1022
Abstract
Comparative analysis can provide important insights into complex biological systems. As demonstrated in the accompanying paper, translating ribosome affinity purification (TRAP) permits comprehensive studies of translated mRNAs in genetically defined cell populations after physiological perturbations. To establish the generality of this approach, we present translational profiles for 24 CNS cell populations and identify known cell-specific and enriched transcripts for each population. We report thousands of cell-specific mRNAs that were not detected in whole-tissue microarray studies and provide examples that demonstrate the benefits deriving from comparative analysis. To provide a foundation for further biological and in silico studies, we provide a resource of 16 transgenic mouse lines, their corresponding anatomic characterization, and translational profiles for cell types from a variety of central nervous system structures. This resource will enable a wide spectrum of molecular and mechanistic studies of both well-known and previously uncharacterized neural cell populations.
Figures
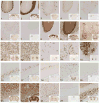
DAB immunohistochemistry with anti-EGFP antibody on each mouse line reveals a unique and specific pattern of expression for the EGFP-L10a transgene. Panels (10x) show the morphology and localization of cell types expressing the transgene, while inset shows location of panel, for cerebellar (1–9), spinal cord (10), striatal/basal forebrain (11–14) brainstem (15), and cortical (16–25) cell types. A key for all cell types is in Figure 2A. Dashed lines (panels 16–25) indicate corpus callosum.
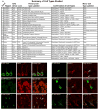
A) All primary cell types expressing EGFP-L10a are listed, as well as the methods used to confirm correct expression. Minor cell types expressing relatively low levels of the EGFP-L10a transgene in the same structure are also listed. Panel # corresponds to Figure 1. B-G) IF on six mouse lines confirms transgene expression in distinct cell types in the cerebellum. First panels show IF for the EGFP-L10a fusion protein (green) in PCP2 (B), NeuroD1 (C), Lypd6 (D), Grm2 (E), Grp (F), and Sept4 (G) BACarray lines. Second panels (red) show co-staining with appropriate cell-type specific markers: Calbindin positive Purkinje cells (B), NeuN positive granule cells (C), Parvalbumin positive outer stellate and deep stellate (basket) neurons of the molecular layer (D), Grm2/3 positive interneurons (Golgi cells) (E), unipolar brush cells with Grm1 positive brush (arrow) (F), and S100 positive Bergman glia (G). The third panels show merged images combining EGFP-L10a and cell-type markers. Note that EGFP-L10a is not detected in the parvalbumin positive Purkinje cells of the Lypd6 line (D, arrow), nor in the glomeruli of the Grm2 line (E, arrow).
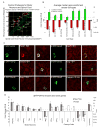
A) Known markers of spinal cord motor neurons (green dots) are highly enriched in the BACarray RNA (IP) (X-axis) while non-motor neuron genes (glial genes, red dots) are enriched in the whole tissue (UB) RNA. B) Averages of known cell-specific markers (green bars) are consistently enriched in the IP RNA, while negative controls (red bars) are not. Exceptions are the mature oligodendrocytes (Cmtm5) with low transgene expression, and granule cells (Neurod1) which contribute the majority of the cerebellar UB RNA thus precluding enrichment. C) ISH (red) and IF (green) images for genes predicted to be expressed in cerebellar Golgi cells show five of six genes with clear double labeling. D) qRT-PCR confirms that the sixth gene, Ceacam10, is expressed in the cerebellum and enriched in Golgi cells. qRT-PCR also confirms BACarray data for genes in Motor Neurons and Purkinje Cells. Slc18a3, Chat, Gfap, Pcp2, and Cnp are positive and negative controls for these populations. * Crygs and Tpm2 failed to amplify by RT-PCR for either IP (Tpm2) or UB (Crygs), and thus no ratio could be calculated. All plots Mean +/− SEM.
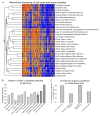
A) Hierarchical clustering on high coefficient of variation genes from all samples describes the relationships between cell types. B) Counting detectable (signal >50) probesets in cerebellar samples reveals that while fewer probesets will be detected in any given cell type than are detectable in whole tissue, across all cell types in total, more probesets have measurable signal. Data normalized to number of probesets in whole cerebellum. C) For four representative cell types, up to 42% of cell-specific or enriched probesets (IP/UB >2) are undetectable on whole tissue microarrays.
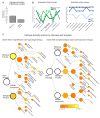
A) Shannon entropy analysis reveals that BACarray samples have twice as much information as whole tissue (Mean +/− SEM). B) Binned expression of probesets for three genes with high Shannon entropy (average entropy 1.68) or low entropy (average entropy .31) shown for eight representative cell types. C) Gene Ontologies analysis identifies significantly over-represented (p<.001) gene classifications for the 10% of probesets with the highest (left panel) or the 10% with lowest (right panel), information content. Color bar: significance level for categories by Hypergeometic test with Benjamini Hochberg FDR correction.
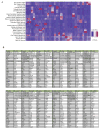
A) Heatmap showing the normalized expression of the 100 top ranked probesets from each sample, across all samples. Note blocks of genes detected as specific to each cell type (such as Pcp2). Related cell types are evidenced by co-expression of some of these genes (such as Bergman glia, and cerebellar astrocytes). B) Lists of the top 25 probesets of the 100 for each cell population from A include many known cell specific genes (for example Pcp2 and Calb1 in Purkinje cells), as well as a variety of novel genes and probesets (such as 2410124H12Rik). Columns are headed with the tissue source (Striatum: Str; Cerebellum: Cb; Cortex: Ctx; Spinal Cord: SC; Brain Stem: BrSt; Basal Forebrain: BF; and Corpus Striatum: CorpStr) as well as the appropriate BACarray driver. Column order corresponds to cell type order in A.
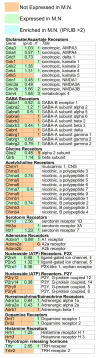
Data from MN BACarrays was directly compared to available data for classical neurotransmitters. To perform this analysis, BACarray results were color coded as ‘expressed,’ ‘enriched,’ or ‘not expressed’. This classification was then compared to results reported in the adult rodent literature, color coded simply as either ‘expressed’ or ‘not expressed’ or left uncolored in cases where there were no studies or conflicting data. IP/UB: Fold change versus whole spinal cord for expressed genes. RF: Expression data from published rodent literature. 1 (Rekling et al., 2000), 2 (Nishi et al., 2001), 3 (Berthele et al., 1999), 4 (Towers et al., 2000), 5 (Malosio et al., 1991), 6 (Kaelin-Lang et al., 1999).
Comment in
-
Unlocking CNS cell type heterogeneity.
Emery B, Barres BA. Emery B, et al. Cell. 2008 Nov 14;135(4):596-8. doi: 10.1016/j.cell.2008.10.031. Cell. 2008. PMID: 19013270
Similar articles
-
A translational profiling approach for the molecular characterization of CNS cell types.
Heiman M, Schaefer A, Gong S, Peterson JD, Day M, Ramsey KE, Suárez-Fariñas M, Schwarz C, Stephan DA, Surmeier DJ, Greengard P, Heintz N. Heiman M, et al. Cell. 2008 Nov 14;135(4):738-48. doi: 10.1016/j.cell.2008.10.028. Cell. 2008. PMID: 19013281 Free PMC article.
-
Rapid Molecular Profiling of Defined Cell Types Using Viral TRAP.
Nectow AR, Moya MV, Ekstrand MI, Mousa A, McGuire KL, Sferrazza CE, Field BC, Rabinowitz GS, Sawicka K, Liang Y, Friedman JM, Heintz N, Schmidt EF. Nectow AR, et al. Cell Rep. 2017 Apr 18;19(3):655-667. doi: 10.1016/j.celrep.2017.03.048. Cell Rep. 2017. PMID: 28423326 Free PMC article.
-
Unlocking CNS cell type heterogeneity.
Emery B, Barres BA. Emery B, et al. Cell. 2008 Nov 14;135(4):596-8. doi: 10.1016/j.cell.2008.10.031. Cell. 2008. PMID: 19013270
-
Wolahan SM, Hirt D, Glenn TC. Wolahan SM, et al. In: Kobeissy FH, editor. Brain Neurotrauma: Molecular, Neuropsychological, and Rehabilitation Aspects. Boca Raton (FL): CRC Press/Taylor & Francis; 2015. Chapter 25. In: Kobeissy FH, editor. Brain Neurotrauma: Molecular, Neuropsychological, and Rehabilitation Aspects. Boca Raton (FL): CRC Press/Taylor & Francis; 2015. Chapter 25. PMID: 26269925 Free Books & Documents. Review.
-
Characterization of mRNA expression in single neurons.
Lin DM, Loveall B, Ewer J, Deitcher DL, Sucher NJ. Lin DM, et al. Methods Mol Biol. 2007;399:133-52. doi: 10.1007/978-1-59745-504-6_10. Methods Mol Biol. 2007. PMID: 18309930 Review.
Cited by
-
Biphasic mechanisms of neurovascular unit injury and protection in CNS diseases.
Maki T, Hayakawa K, Pham LD, Xing C, Lo EH, Arai K. Maki T, et al. CNS Neurol Disord Drug Targets. 2013 May 1;12(3):302-15. doi: 10.2174/1871527311312030004. CNS Neurol Disord Drug Targets. 2013. PMID: 23469847 Free PMC article. Review.
-
Mitsi V, Ruiz A, Polizu C, Farzinpour Z, Ramakrishnan A, Serafini RA, Parise EM, Floodstrand M, Sial OK, Gaspari S, Tang CY, Nestler EJ, Schmidt EF, Shen L, Zachariou V. Mitsi V, et al. Mol Pharmacol. 2024 Mar 14;105(4):272-285. doi: 10.1124/molpharm.123.000753. Mol Pharmacol. 2024. PMID: 38351270
-
Nousch M. Nousch M. G3 (Bethesda). 2020 Nov 5;10(11):4063-4069. doi: 10.1534/g3.120.401644. G3 (Bethesda). 2020. PMID: 32883755 Free PMC article.
-
RNA protein interaction in neurons.
Darnell RB. Darnell RB. Annu Rev Neurosci. 2013 Jul 8;36:243-70. doi: 10.1146/annurev-neuro-062912-114322. Epub 2013 May 20. Annu Rev Neurosci. 2013. PMID: 23701460 Free PMC article. Review.
-
Selective Neuronal Vulnerability in Alzheimer's Disease: A Network-Based Analysis.
Roussarie JP, Yao V, Rodriguez-Rodriguez P, Oughtred R, Rust J, Plautz Z, Kasturia S, Albornoz C, Wang W, Schmidt EF, Dannenfelser R, Tadych A, Brichta L, Barnea-Cramer A, Heintz N, Hof PR, Heiman M, Dolinski K, Flajolet M, Troyanskaya OG, Greengard P. Roussarie JP, et al. Neuron. 2020 Sep 9;107(5):821-835.e12. doi: 10.1016/j.neuron.2020.06.010. Epub 2020 Jun 29. Neuron. 2020. PMID: 32603655 Free PMC article.
References
-
- Bargmann CI. Genetic and cellular analysis of behavior in C. elegans. Annu Rev Neurosci. 1993;16:47–71. - PubMed
-
- Berthele A, Boxall SJ, Urban A, Anneser JM, Zieglgansberger W, Urban L, Tolle TR. Distribution and developmental changes in metabotropic glutamate receptor messenger RNA expression in the rat lumbar spinal cord. Brain Res Dev Brain Res. 1999;112:39–53. - PubMed
-
- Blais A, Dynlacht BD. Constructing transcriptional regulatory networks. Genes Dev. 2005;19:1499–1511. - PubMed
-
- Fuhrman S, Cunningham MJ, Wen X, Zweiger G, Seilhamer JJ, Somogyi R. The application of shannon entropy in the identification of putative drug targets. Biosystems. 2000;55:5–14. - PubMed
Publication types
MeSH terms
Substances
Grants and funding
- P01 AG009464-16A1/AG/NIA NIH HHS/United States
- P50 MH074866-03/MH/NIMH NIH HHS/United States
- P50 MH074866-029001/MH/NIMH NIH HHS/United States
- P50 MH074866/MH/NIMH NIH HHS/United States
- P50 MH074866-020001/MH/NIMH NIH HHS/United States
- HHMI/Howard Hughes Medical Institute/United States
- P50 MH074866-030001/MH/NIMH NIH HHS/United States
- P50 MH074866-029002/MH/NIMH NIH HHS/United States
- P50 MH074866-010001/MH/NIMH NIH HHS/United States
- P50 MH074866-039002/MH/NIMH NIH HHS/United States
- P01 DA010044-11/DA/NIDA NIH HHS/United States
- P50 MH074866-049001/MH/NIMH NIH HHS/United States
- P50 MH074866-020002/MH/NIMH NIH HHS/United States
- P50 MH074866-019002/MH/NIMH NIH HHS/United States
- F32 DA021487-01/DA/NIDA NIH HHS/United States
- P50 MH074866-030002/MH/NIMH NIH HHS/United States
- 5F32DA021487/DA/NIDA NIH HHS/United States
- P50 MH074866-019001/MH/NIMH NIH HHS/United States
- P50 MH074866-02/MH/NIMH NIH HHS/United States
- P50 MH074866-040005/MH/NIMH NIH HHS/United States
- P01 AG009464/AG/NIA NIH HHS/United States
- P50 MH074866-030005/MH/NIMH NIH HHS/United States
- P01 AG009464-17/AG/NIA NIH HHS/United States
- P50 MH074866-020005/MH/NIMH NIH HHS/United States
- P50 MH074866-010005/MH/NIMH NIH HHS/United States
- P01 DA010044/DA/NIDA NIH HHS/United States
- MH074866/MH/NIMH NIH HHS/United States
- P50 MH074866-039001/MH/NIMH NIH HHS/United States
- F32 DA021487-02/DA/NIDA NIH HHS/United States
- AG09464/AG/NIA NIH HHS/United States
- P50 MH074866-040001/MH/NIMH NIH HHS/United States
- P50 MH074866-049002/MH/NIMH NIH HHS/United States
- P50 MH074866-010002/MH/NIMH NIH HHS/United States
- P50 MH074866-04/MH/NIMH NIH HHS/United States
- P50 MH074866-040002/MH/NIMH NIH HHS/United States
- F32 DA021487/DA/NIDA NIH HHS/United States
- P01 DA010044-10/DA/NIDA NIH HHS/United States
- P01 DA010044-09/DA/NIDA NIH HHS/United States
- P50 MH074866-017204/MH/NIMH NIH HHS/United States
- DA10044/DA/NIDA NIH HHS/United States
LinkOut - more resources
Full Text Sources
Other Literature Sources
Molecular Biology Databases
Research Materials