Cohesin associates with spindle poles in a mitosis-specific manner and functions in spindle assembly in vertebrate cells - PubMed
Cohesin associates with spindle poles in a mitosis-specific manner and functions in spindle assembly in vertebrate cells
Xiangduo Kong et al. Mol Biol Cell. 2009 Mar.
Abstract
Cohesin is an essential protein complex required for sister chromatid cohesion. Cohesin associates with chromosomes and establishes sister chromatid cohesion during interphase. During metaphase, a small amount of cohesin remains at the chromosome-pairing domain, mainly at the centromeres, whereas the majority of cohesin resides in the cytoplasm, where its functions remain unclear. We describe the mitosis-specific recruitment of cohesin to the spindle poles through its association with centrosomes and interaction with nuclear mitotic apparatus protein (NuMA). Overexpression of NuMA enhances cohesin accumulation at spindle poles. Although transient cohesin depletion does not lead to visible impairment of normal spindle formation, recovery from nocodazole-induced spindle disruption was significantly impaired. Importantly, selective blocking of cohesin localization to centromeres, which disrupts centromeric sister chromatid cohesion, had no effect on this spindle reassembly process, clearly separating the roles of cohesin at kinetochores and spindle poles. In vitro, chromosome-independent spindle assembly using mitotic extracts was compromised by cohesin depletion, and it was rescued by addition of cohesin that was isolated from mitotic, but not S phase, cells. The combined results identify a novel spindle-associated role for human cohesin during mitosis, in addition to its function at the centromere/kinetochore regions.
Figures
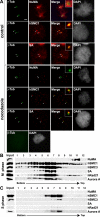
Cohesin association with centrosomes. (A) Microtubule-independent recruitment of cohesin to spindle poles. HeLa cells were treated with 0.1 μg/ml nocodazole for 1 h before preextraction and fixation. Cells were then stained with antibodies specific for γ-tubulin, β-tubulin, NuMA, SMC1, and SA antibodies as indicated. Untreated control mitotic cells are also shown. Bar, 5 μm. The exposure time for all images was identical, with the exception of NuMA in the control cell, which was decreased to one-fifth due to the strong signal. (B) Western blot analysis of the 40–70% sucrose gradient fractions of purified centrosomes from HeLa cells synchronized to mitotic (M) phase. Centrosome peak fractions were detected by the presence of Aurora A. (C) Same as in B, except for S phase-synchronized cells.
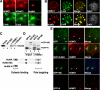
NuMA recruits cohesin to the spindle poles through its N-terminus domain. (A) Cohesin localization to in vitro assembled mitotic spindle asters. Immunofluorescent staining of in vitro assembled mitotic spindle asters with mAb specific for β-tubulin (β-tub) and polyclonal antibodies specific for SMC1, Rad21, or NuMA as indicated. Bar, 2 μm. (B) Cohesin localization to Taxol-induced centrosome-independent asters in vivo. HeLa cells were treated with nocodazole and Taxol sequentially followed by preextraction and fixation. Cells were then stained with antibodies specific for β-tubulin, SMC1, and NuMA as indicated. Bar, 5 μm. (C) Coimmunoprecipitation-Western analysis of the interaction between endogenous cohesin and GFP-NuMA in vivo. Extracts from 293T cells expressing the full-length NuMA fused to GFP (lanes 1–4) were used for coimmunoprecipitation with antibody against SMC1 (αSMC1; lane 3), Rad21 (αRad21; lanes 4), or preimmune IgG (pre-IgG; lane 2), and eluted with 2 M guanidine-HCl after a 1 M KCl wash. Anti-GFP antibody was used as the primary antibody for Western analysis. (D) Coimmunoprecipitation-Western analysis of the interaction between GFP-NuMA deletion mutants and endogenous cohesin. The experiments were carried out in a manner similar to C by using anti-hSMC1 antibody for immunoprecipitation and anti-GFP antibody for Western analysis. An asterisk indicates the IgG heavy chain. A schematic diagram of NuMA deletion mutants is shown. The C-terminal spindle pole-targeting domain of NuMA is indicated. (E) The effects of overexpression of the full-length and deletion mutants of GFP-NuMA on cohesin targeting to the spindle poles in vivo. A GFP-only control is also shown. Cells were preextracted before fixation and immunostained with anti-SMC1 antibody. Bar, 5 μm.
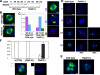
Effects of Rad21 depletion on mitotic spindle organization in DT40 cells. (A) Western analysis of Rad21 conditional knockout [Rad21(−)] chicken DT40 cells after Dox treatment. The wild-type and Rad21(−) cells were treated with Dox for the duration of time indicated at top. (B) Spindle morphology of the wild-type and mutant cells after 24-h Dox treatment. Dox-treated wild-type and Rad21(−) mitotic cells were fixed and stained with antibody specific for α-tubulin (green) and NuMA (red) as well as 4,6-diamidino-2-phenylindole (DAPI; blue). The merged images are shown. Bar, 5 μm. (C) Rad21 depletion affects the efficiency of spindle reassembly. Wild-type or Rad21(−) cells were treated with nocodazole for 1 h after 24-h incubation with Dox. Cells were then released from nocodazole treatment and incubated for 1 h to restore the spindle. Immunostaining is same as described in B. The percentage of normal and abnormal spindles is shown in the graph. The total numbers of mitotic cells examined are shown at the bottom. Representative spindle morphology for each category (specifically, “normal spindles,” “abnormal short spindles,” and “poles with no visible asters”) is shown. Bar, 5 μm. (D) Time course analysis of spindle reassembly in Rad21(−) DT40 cells after nocodazole release. Spindle morphology in mitotic cells was analyzed at 1, 5, 10, and 15 min after nocodazole release as indicated. Representative morphology of spindles is shown. Green, α-tubulin; red, NuMA; and blue, DAPI. Almost no spindle was observed within the first 15 min of reassembly in 70% of the Rad21-depleted mitotic cells examined, in contrast to the efficient recovery of spindles in the wild-type cells. The other 30% of mutant mitotic cells exhibited shorter spindles compared with wild type cells. Bar, 5 μm. (E) Rad21 depletion inhibits Taxol-induced nonspecific aster assembly in vivo. After the nocodazole treatment as described in C, wild-type or Rad21(−) cells were further incubated with Taxol. Cells were then fixed and stained with anti-α-tubulin antibody and DAPI. The wild-type cells exhibited centrosome-independent aster formation upon Taxol treatment, whereas Rad21-depleted cells failed to form asters. Bar, 5 μm. (F) Prolonged Rad21 depletion abolishes mitotic spindle formation. Rad21(−) cells were treated with Dox for 27 h, and mitotic spindle morphology was compared with Dox-treated wild type cells and CENP-H(−) cells. Approximately 40 mitotic cells for each cell line were analyzed in one experiment and the experiments were repeated three times to obtain the SE of the mean. The percentage of mitotic cells with and without spindles is shown in the graph. Representative images (“spindle-positive” and “poles with no visible asters”) (staining is the same as described in B) is shown below.
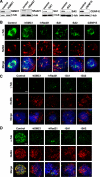
Cohesin depletion inhibits mitotic spindle recovery in vivo. (A) Western blot analysis of siRNA-transfected cell lysates. Cell lysates were prepared 24 h after the second transfection with siRNA for hSMC1, Rad21, SA1, SA2, or CENP-E. Control cells were transfected with a randomized siRNA. β-Tubulin serves as a loading control. (B) Cohesin depletion inhibits the spindle recovery. Cells transfected with siRNA were treated with nocodazole and incubated in fresh medium for 20 min before fixation. Mitotic cells were stained with antibodies specific for α-tubulin (green) and NuMA (red). DNA is visualized by DAPI staining (blue). The depleted proteins are indicated at top. Bar, 5 μm. (C) Multiple NuMA signals are not due to centrosome amplification. In a similar experiment as described in B, cells were stained with γ-tubulin (green) to visualize centrosomes and colocalization with NuMA was analyzed. Bar, 5 μm. (D) Cohesin depletion inhibits Taxol-induced spindle asters in vivo. Cohesin-depleted cells and control cells were treated with Taxol for 5 min as in Figure 2B. Bar, 5 μm.
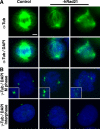
hRad21 depletion induces spindle abnormality and centrosome fragmentation. (A) Spindle morphology of control- and hRad21-siRNA transfected cells. For prolonged Rad21 depletion, siRNA transfection was repeated three times at 24-h intervals. At 12 h after the third siRNA transfection, hRad21 depletion led to spindle abnormality in ∼40% of mitotic cells. Top, α-tubulin only. Bottom, α-tubulin and DAPI overlay. Bar, 5 μm. (B) γ-Tubulin and DAPI staining of mitotic and interphase cells. M phase centrosomes in high magnification are shown. Magnification is identical to that described in A.
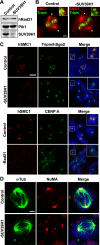
Depletion of SUV39H1 selectively inhibits cohesin function at centromeres but not at spindle poles. (A) Western blot analysis of control- and SUV39H1-siRNA–treated cells. There is no effect on the cohesin subunit hRad21. Plk1 serves as a loading control. (B) Cohesin localization at kinetochores, but not spindle poles, is affected by SUV39H1 depletion. Cells growing on glass coverslips were extracted with MTSB buffer (MTSB: 4 M glycerol, 100 mM PIPES, pH 6.8, 1 mM EGTA, and 5 mM MgCl2) containing 0.5% Triton X-100 for 2 min at room temperature followed by rinsing in MTSB. They were then fixed in −20°C methanol for 10 min. Cohesin is detected by antibody specific for hSMC1 (red). Antibody specific for Tripin/hSgo2 (green) was used as a centromere marker. The kinetochore regions in higher magnification are shown at the top right corners. Spindle poles are indicated by arrows. Bar, 5 μm. (C) Metaphase chromosome spreads of control- and SUV39H1-siRNA–treated cells. Immunofluorescent staining was carried out using antibodies specific for hSMC1 (red) and Tripin/Sgo2 (green). For comparison, metaphase chromosome spreads of control- and Rad21-depleted cells are also shown using antibodies specific for hSMC1 (red) and CENP-A (green). DNA is visualized by DAPI staining (blue). Bar, 2 μm. The kinetochore regions in higher magnification are shown at the top right corners. (D) SUV39H1 depletion does not affect spindle recovery. Cells transfected with siRNA as indicated were treated with nocodazole and incubated in fresh medium for 20 min before fixation similar to the experiments in Figure 4B. Mitotic cells were stained with antibodies specific for α-tubulin (green) and NuMA (red) as indicated at top. Bar, 5 μm.
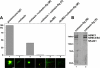
M phase-, but not S phase-, cohesin complements mitotic spindle aster assembly in vitro. Bar, 2 μm. (A) Cohesin add-back to cohesin- and NuMA-depleted extracts. Cohesin was depleted from HeLa mitotic aster assembly extracts using anti-Rad21 antibody (-cohesin, lanes 2–4). Aster assembly was compared with those of the preimmune IgG-depleted extracts (lane 1), and the extracts depleted with anti-NuMA antibody (-NuMA, lanes 5 and 6). Lane 3, FLAG-purified cohesin (cohesin-flg) from M phase extracts was added to the cohesin-depleted extracts before aster assembly. Lane 4, a comparable amount of cohesin-flg purified from S phase-synchronized extracts was added to the cohesin-depleted extracts. Lane 6, M phase cohesin-flg was added to the NuMA-depleted extracts before aster assembly. Typical spindle morphology observed in each depletion (and add-back) is shown below. Green, β-tubulin. Red, NuMA. Yellow, overlap between β-tubulin and NuMA. (B) A silver stain of cohesin-flg from S phase and M phase. Cohesin components are indicated.
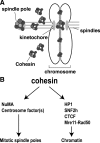
A model for two distinct roles of cohesin in mitotic chromosome organization and function. (A) During mitosis, cohesin has two distinct functions. By associating with chromosomes, cohesin mediates the pairing of sister chromatids. By associating with mitotic spindle poles, cohesin plays a role in mitotic spindle dynamics. (B) The functional specificities of cohesin at different subcellular locations may be determined by the interactions with factors specific for each role. For example, HP1, SNF2h, CTCF, and Mre11-Rad50 were shown to function in chromosome loading of cohesin, whereas NuMA and an unknown centrosome component(s) are required for its spindle pole localization in vertebrate cells.
Similar articles
-
A potential role for human cohesin in mitotic spindle aster assembly.
Gregson HC, Schmiesing JA, Kim JS, Kobayashi T, Zhou S, Yokomori K. Gregson HC, et al. J Biol Chem. 2001 Dec 14;276(50):47575-82. doi: 10.1074/jbc.M103364200. Epub 2001 Oct 4. J Biol Chem. 2001. PMID: 11590136
-
Essential roles for cohesin in kinetochore and spindle function in Xenopus egg extracts.
Kenney RD, Heald R. Kenney RD, et al. J Cell Sci. 2006 Dec 15;119(Pt 24):5057-66. doi: 10.1242/jcs.03277. J Cell Sci. 2006. PMID: 17158911
-
Toyoda Y, Yanagida M. Toyoda Y, et al. Mol Biol Cell. 2006 May;17(5):2287-302. doi: 10.1091/mbc.e05-11-1089. Epub 2006 Mar 1. Mol Biol Cell. 2006. PMID: 16510521 Free PMC article.
-
An update on cohesin function as a 'molecular glue' on chromosomes and spindles.
Wong RW. Wong RW. Cell Cycle. 2010 May;9(9):1754-8. doi: 10.4161/cc.9.9.11806. Epub 2010 May 18. Cell Cycle. 2010. PMID: 20436296 Review.
-
Sister chromatid cohesion and genome stability in vertebrate cells.
Morrison C, Vagnarelli P, Sonoda E, Takeda S, Earnshaw WC. Morrison C, et al. Biochem Soc Trans. 2003 Feb;31(Pt 1):263-5. doi: 10.1042/bst0310263. Biochem Soc Trans. 2003. PMID: 12546698 Review.
Cited by
-
Separate to operate: control of centrosome positioning and separation.
Agircan FG, Schiebel E, Mardin BR. Agircan FG, et al. Philos Trans R Soc Lond B Biol Sci. 2014 Sep 5;369(1650):20130461. doi: 10.1098/rstb.2013.0461. Philos Trans R Soc Lond B Biol Sci. 2014. PMID: 25047615 Free PMC article. Review.
-
Sensors at centrosomes reveal determinants of local separase activity.
Agircan FG, Schiebel E. Agircan FG, et al. PLoS Genet. 2014 Oct 9;10(10):e1004672. doi: 10.1371/journal.pgen.1004672. eCollection 2014 Oct. PLoS Genet. 2014. PMID: 25299182 Free PMC article.
-
Liu C, Shi Y, Li J, Liu X, Xiahou Z, Tan Z, Chen X, Li J. Liu C, et al. J Biol Chem. 2020 May 22;295(21):7341-7349. doi: 10.1074/jbc.RA119.012401. Epub 2020 Apr 15. J Biol Chem. 2020. PMID: 32295844 Free PMC article.
-
Cohesin subunit RAD21: From biology to disease.
Cheng H, Zhang N, Pati D. Cheng H, et al. Gene. 2020 Oct 20;758:144966. doi: 10.1016/j.gene.2020.144966. Epub 2020 Jul 17. Gene. 2020. PMID: 32687945 Free PMC article. Review.
-
Haspin: a newly discovered regulator of mitotic chromosome behavior.
Higgins JM. Higgins JM. Chromosoma. 2010 Apr;119(2):137-47. doi: 10.1007/s00412-009-0250-4. Epub 2009 Dec 8. Chromosoma. 2010. PMID: 19997740 Free PMC article. Review.
References
-
- Aagaard L., Schmid M., Warburton P., Jenuwein T. Mitotic phosphorylation of SUV39H1, a novel component of active centromeres, coincides with transient accumulation at mammalian centromeres. J. Cell Sci. 2000;113:817–829. - PubMed
-
- Bernard P., Maure J.-F., Partridge J. F., Genier S., Javerzat J.-P., Allshire R. C. Requirement of heterochromatin for cohesion at centromeres. Science. 2001;294:2539–2542. - PubMed
-
- Blower M. D., Nachury M., Heald R., Weis K. A Rae1-containing ribonucleoprotein complex is required for mitotic spindle assembly. Cell. 2005;121:223–234. - PubMed
Publication types
MeSH terms
Substances
Grants and funding
LinkOut - more resources
Full Text Sources
Research Materials
Miscellaneous