The electrotonic structure of pyramidal neurons contributing to prefrontal cortical circuits in macaque monkeys is significantly altered in aging - PubMed
The electrotonic structure of pyramidal neurons contributing to prefrontal cortical circuits in macaque monkeys is significantly altered in aging
Doron Kabaso et al. Cereb Cortex. 2009 Oct.
Abstract
Whereas neuronal numbers are largely preserved in normal aging, subtle morphological changes occur in dendrites and spines, whose electrotonic consequences remain unexplored. We examined age-related morphological alterations in 2 types of pyramidal neurons contributing to working memory circuits in the macaque prefrontal cortex (PFC): neurons in the superior temporal cortex forming "long" projections to the PFC and "local" projection neurons within the PFC. Global dendritic mass homeostasis, measured by 3-dimensional scaling analysis, was conserved with aging in both neuron types. Spine densities, dendrite diameters, lengths, and branching complexity were all significantly reduced in apical dendrites of long projection neurons with aging, but only spine parameters were altered in local projection neurons. Despite these differences, voltage attenuation due to passive electrotonic structure, assuming equivalent cable parameters, was significantly reduced with aging in the apical dendrites of both neuron classes. Confirming the electrotonic analysis, simulated passive backpropagating action potential efficacy was significantly higher in apical but not basal dendrites of old neurons. Unless compensated by changes in passive cable parameters, active membrane properties, or altered synaptic properties, these effects will increase the excitability of pyramidal neurons, compromising the precisely tuned activity required for working memory, ultimately resulting in age-related PFC dysfunction.
Figures
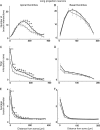
The 3-D Sholl analyses of long projection neurons from young (black lines) and old (gray lines) macaque monkeys in apical (A, C, E) and basal dendrites (B, D, F). Asterisks indicate statistically significant differences across the dendritic tree (*P < 0.05; **P < 0.01). Values represent means ± standard error of the mean.
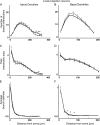
The 3-D Sholl analyses of local projection neurons from young (black lines) and old (gray lines) macaque monkeys in apical (A, C, E) and basal dendrites (B, D, F). Asterisks indicate statistically significant differences across the dendritic tree (*P < 0.05). Values represent means ± standard error of the mean.
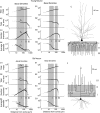
Optimized fits of scaling exponents (black fitted lines, A3, A4; D3, D4) and optimal scaling regions (gray shaded bands) for typical young and old long projection neurons. Scaling exponents (dM, dA, dN, and dT) are fitted on a log-log scale to cumulative mass (A1, B1, D1, E1), total area (A2, B2, D2, E2), number of branches (A3, B3, D3, E3), and average diameter (A4, B4, D4, E4) of the intersections with distance from the soma over the proximal (I) and medial (II) regions of basal and apical dendrites. Representative linear fits of dN and dT (black lines) in Region I are shown for the apical trees in the young neuron (A3 and A4) and the old neuron (D3 and D4). Two-dimensional projections of 3-D reconstructed young (C) and old (F) neurons from which the log-log plots were measured. (C) The basal tree of the young neuron is represented in dendrogram space, whereas the apical dendrites remain in anatomical space. (F) The apical tree of the old neuron is represented in dendrogram space, whereas the basal dendrites remain in anatomical space. Total int. area: total cross-sectional intersection area of branches with sequential parallel planes in dendrogram space. I: proximal scaling region; II: medial scaling region; and III: distal nonscaling die-off region.
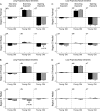
Contributions of branching and tapering exponents to global mass scaling (measured by total area exponent, dA) in spine-corrected apical (A) and basal (B) dendrites of long projection neurons and in apical (C) and basal (D) dendrites of local projection neurons. The 2 rows of panels (A–D) contrast the different branching and tapering patterns in Region I (top rows, A–D) with Region II (bottom rows, A–D). Means ± standard error of the mean are represented as histograms (black, young; gray, old) with superimposed error bars. The asterisks in (A, B) indicate statistically significant (P < 0.05) age-related reductions in tapering and branching exponent of long projection neurons.
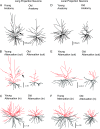
Inward and outward morphoelectrotonic transforms of representative young and old spine-corrected long (A–C) and local (D–F) projection neurons at 0 Hz. The top row shows anatomical reconstructions of young and old long (A) and local (D) projection neurons, plotted on the same physical length scales for comparison (black bar representing 100 μm in A, D). The outward attenuation transforms of these neurons are shown in the second row (B, E), plotted on the same length scale in attenuation space (L = 0.1; black bar). Inward transforms are in the third row (C, F). In each of the 3 rows, transformed neurons are plotted on the same scale for comparison. Note the increase in scale for inward attenuation transforms (L = 1.0; black bar in C, F) compared with outward attenuation transforms (B, E). Transformed apical dendrites are shown in red to aid visualization. Individual dendritic sections are affected differently by the transform: examples of relatively larger (black) and smaller (gray) sections are pointed out with arrows in (A, B).
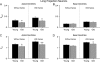
Effects of aging on mean inward (in) and outward (
out) attenuation transforms at DC in long projection neurons. Histograms show means ± standard error of the mean (error bars) of
out (A, B) and
in (C, D) for the young (black bars) and old (gray bars) neuron groups. In each cell (A–D), mean attenuation transforms were compared for models without spines (left panels) or including spines (right panels). Asterisks indicate statistically significant differences (*P < 0.05; **P < 0.01). Values represent means ± standard error of the mean.
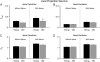
Effects of aging on mean inward (in) and outward (
out) attenuation transforms at DC in local projection neurons. Format as in Figure 6.
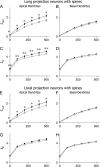
Effect of increasing frequency (0–500 Hz) on in and
out transforms in young (solid black line) and old (dashed lines) neurons. Plotted points at each 100 Hz interval represent means ± standard error of the mean. Asterisks indicate statistically significant differences (*P < 0.05; **P < 0.01). Data series in (H) were virtually identical: aged neuron data have been shifted downward by a value of 0.1 on the log attenuation axis to aid visualization.
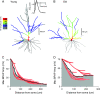
Simulated passive AP backpropagation in apical dendrites of representative young (A) and old (B) long projection neurons. (A, B) Reconstructed morphology of each cell is shown above, with superimposed color map representing peak voltage (relative to resting potential of −65 mV) attained by the backpropagating AP injected under voltage clamp at the soma, according to the color scale shown at left. The somatic AP is shown in the inset (A). (C, D) Maximum backpropagating AP amplitudes (relative to −65 mV) attained in each apical branch segment (red curves) are plotted against the distance in dendrogram space from the soma for young (C) and old (D) neurons. The black curve describes the mean maximum backpropagating AP amplitude computed at each distance from the soma. Normalized backpropagation area (NBParea, shown as gray region) was computed as described in the text.
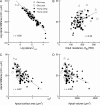
Comparison of traditional morphometric measures, total surface area and total volume, as predictors of backpropagation efficacy (NBParea), with electrotonic measures. NBParea is plotted as a scatterplot versus each of the predictive variables, and the best-fitting first-order regression line superimposed. (A) The electrotonic measure out is the best predictor of NBParea, with a strong linear negative correlation that becomes even stronger on a log-log scale. (B) Input resistance at the base of the apical trunk, RN, showed a moderate, positive correlation; (C) total surface area showed a moderate, negative correlation; and (D) total volume was poorly correlated with backpropagation efficacy.
Similar articles
-
Duan H, Wearne SL, Morrison JH, Hof PR. Duan H, et al. Neuroscience. 2002;114(2):349-59. doi: 10.1016/s0306-4522(02)00305-6. Neuroscience. 2002. PMID: 12204204
-
Age-related dendritic and spine changes in corticocortically projecting neurons in macaque monkeys.
Duan H, Wearne SL, Rocher AB, Macedo A, Morrison JH, Hof PR. Duan H, et al. Cereb Cortex. 2003 Sep;13(9):950-61. doi: 10.1093/cercor/13.9.950. Cereb Cortex. 2003. PMID: 12902394
-
Functionally relevant measures of spatial complexity in neuronal dendritic arbors.
Rothnie P, Kabaso D, Hof PR, Henry BI, Wearne SL. Rothnie P, et al. J Theor Biol. 2006 Feb 7;238(3):505-26. doi: 10.1016/j.jtbi.2005.06.001. Epub 2005 Aug 3. J Theor Biol. 2006. PMID: 16083911
-
Yang CR, Seamans JK, Gorelova N. Yang CR, et al. Neuropsychopharmacology. 1999 Aug;21(2):161-94. doi: 10.1016/S0893-133X(98)00112-2. Neuropsychopharmacology. 1999. PMID: 10432466 Review.
-
Effects of normal aging on prefrontal area 46 in the rhesus monkey.
Luebke J, Barbas H, Peters A. Luebke J, et al. Brain Res Rev. 2010 Mar;62(2):212-32. doi: 10.1016/j.brainresrev.2009.12.002. Epub 2009 Dec 11. Brain Res Rev. 2010. PMID: 20005254 Free PMC article. Review.
Cited by
-
Chang W, Weaver CM, Medalla M, Moore TL, Luebke JI. Chang W, et al. Neurobiol Aging. 2022 Jan;109:113-124. doi: 10.1016/j.neurobiolaging.2021.09.012. Epub 2021 Sep 16. Neurobiol Aging. 2022. PMID: 34715442 Free PMC article.
-
Rodriguez-Rodriguez P, Arroyo-Garcia LE, Tsagkogianni C, Li L, Wang W, Végvári Á, Salas-Allende I, Plautz Z, Cedazo-Minguez A, Sinha SC, Troyanskaya O, Flajolet M, Yao V, Roussarie JP. Rodriguez-Rodriguez P, et al. Brain. 2024 Jul 5;147(7):2384-2399. doi: 10.1093/brain/awae051. Brain. 2024. PMID: 38462574 Free PMC article.
-
López-Doménech G, Higgs NF, Vaccaro V, Roš H, Arancibia-Cárcamo IL, MacAskill AF, Kittler JT. López-Doménech G, et al. Cell Rep. 2016 Oct 4;17(2):317-327. doi: 10.1016/j.celrep.2016.09.004. Cell Rep. 2016. PMID: 27705781 Free PMC article.
-
Grydeland H, Vértes PE, Váša F, Romero-Garcia R, Whitaker K, Alexander-Bloch AF, Bjørnerud A, Patel AX, Sederevicius D, Tamnes CK, Westlye LT, White SR, Walhovd KB, Fjell AM, Bullmore ET. Grydeland H, et al. Cereb Cortex. 2019 Mar 1;29(3):1369-1381. doi: 10.1093/cercor/bhy330. Cereb Cortex. 2019. PMID: 30590439 Free PMC article.
-
Zheng DD, Liu ZH, Fang J, Wang XY, Zhang J. Zheng DD, et al. AJNR Am J Neuroradiol. 2012 Mar;33(3):563-8. doi: 10.3174/ajnr.A2793. Epub 2011 Nov 11. AJNR Am J Neuroradiol. 2012. PMID: 22081680 Free PMC article.
References
-
- Bachevalier J, Landis LS, Walker LC, Brickson M, Mishkin M, Price DL, Cork LC. Aged monkeys exhibit behavioral deficits indicative of widespread cerebral dysfunction. Neurobiol Aging. 1991;12:99–111. - PubMed
-
- Barbas H. Pattern in the laminar origin of corticocortical connections. J Comp Neurol. 1986;252:415–422. - PubMed
-
- Brown TH, Zador A, Mainen ZF, Claiborne BJ. Hebbian computations in hippocampal dendrites and spines. In: McKenna T, Davis J, Zornetzer SF, editors. Single neuron computation. San Diego (CA): Academic Press; 1992. pp. 81–116.
-
- Bui TV, Cushing S, Dewey D, Fyffe RE, Rose PK. Comparison of the morphological and electrotonic properties of Renshaw cells, Ia inhibitory interneurons, and motoneurons in the cat. J Neurophysiol. 2003;90:2900–2918. - PubMed
Publication types
MeSH terms
Grants and funding
LinkOut - more resources
Full Text Sources
Medical
Miscellaneous