Unexpected patterns of plastic energy allocation in stochastic environments - PubMed
Unexpected patterns of plastic energy allocation in stochastic environments
Barbara Fischer et al. Am Nat. 2009 Mar.
Abstract
When environmental conditions vary stochastically, individuals accrue fitness benefits by exhibiting phenotypic plasticity. Here we analyze a general dynamic-programming model describing an individual's optimal energy allocation in a stochastic environment. After maturation, individuals repeatedly decide how to allocate incoming energy between reproduction and maintenance. We analyze the optimal fraction of energy invested in reproduction and the resultant degree of plasticity in dependence on environmental variability and predictability. Our analyses reveal unexpected patterns of optimal energy allocation. When energy availability is low, all energy is allocated to reproduction, although this implies that individuals will not survive after reproduction. Above a certain threshold of energy availability, the optimal reproductive investment decreases to a minimum and even vanishes entirely in highly variable environments. With further improving energy availability, optimal reproductive investment gradually increases again. Costs of plasticity affect this allocation pattern only quantitatively. Our results show that optimal reproductive investment does not increase monotonically with growing energy availability and that small changes in energy availability can lead to major variations in optimal energy allocation. Our results help to unify two apparently opposing predictions from life-history theory, that organisms should increase reproductive investment both with improved environmental conditions and when conditions deteriorate ("terminal investment").
Figures
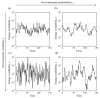
Stochastic fluctuations in energy availability in four environments with different variability and predictability. The average amplitude of the time series increases with environmental variability λ, whereas its average frequency decreases with environmental predictability τ. Dotted lines show the resultant 95% confidence intervals for energy availability. Environmental variability λ is larger in the bottom row (c, d) than it is in the top row (a, b), while environmental predictability τ is larger in the right column (b, d) than it is in the left column (a, c): a, λ = 5, τ = 1; b, λ = 5, τ = 10; c, λ = 50, τ = 1; and d, λ = 50, τ = 10. Note that the vertical axes are scaled logarithmically.
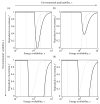
Evolutionarily optimal allocation reaction norms, describing the dependence of the optimal reproductive investment f on energy availability e, in four environments with different variability and predictability (fig. 1). Dotted lines show the resultant 95% confidence intervals for energy availability. Dashed curves show the survival probabilities resulting from the presented reaction norm at different energy availabilities. Environmental variability λ is larger in the bottom row (c, d) than it is in the top row (a, b), while environmental predictability τ is larger in the right column (b, d) than it is in the left column (a, c): a, λ = 10, τ = 20; b, λ = 10, τ = 50; c, λ = 50, τ = 20; and d, λ = 50, τ = 50. Note that the horizontal axes are scaled logarithmically. Other parameters: e1/2 = 5.
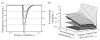
Classification of evolutionarily optimal allocation reaction norms. a, Examples of reaction norms f(e) with minima at f = 0, 0.25, 0.5, or 0.75. b, Surfaces of parameter combinations (e1/2, τ, λ) resulting in optimal reaction norms with these minima. Plasticity thus increases from bottom to top.
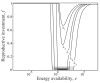
Effects on evolutionarily optimal allocation reaction norms of environmental variability. Optimal reaction norms f(e) are shown for different levels of environmental variability λ: λ1 = 1, λ2 = 10, λ3 =20, λ4 = 50, and λ5 = 100. Increased environmental variability leads to skipped reproduction across wider ranges of energy availability (gray bars). Other parameters: e1/2 = 5 and τ = 50.
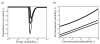
Effects on evolutionarily optimal allocation reaction norms of the scale c of plasticity costs. a, Optimal reaction norms for different values of c, with τ = 50 and λ = 10. Increased plasticity costs reduce the optimal degree of plasticity. b, Lines of parameter combinations (τ, λ) resulting in optimal reaction norms with a minimum f = 0 for different values of c: c = 0, 10, 100, and 1,000 are indicated by growing line widths. Other parameters: e1/2 = 5 and b = 1.
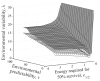
Effects on evolutionarily optimal allocation reaction norms of the proportion b at which plasticity costs affect reproduction as opposed to maintenance. Surfaces of parameter combinations (e1/2, τ, λ) resulting in optimal reaction norms with a minimum at f = 0 for different values of b: b = 0 (white), b = 0.5 (light gray), and b = 1 (dark gray). Increased allocations of plasticity costs to reproduction reduce the optimal degree of plasticity. Other parameters: c = 1.
Similar articles
-
When to store energy in a stochastic environment.
Fischer B, Dieckmann U, Taborsky B. Fischer B, et al. Evolution. 2011 May;65(5):1221-32. doi: 10.1111/j.1558-5646.2010.01198.x. Epub 2010 Dec 22. Evolution. 2011. PMID: 21108636 Free PMC article.
-
The evolution of parental care in stochastic environments.
Bonsall MB, Klug H. Bonsall MB, et al. J Evol Biol. 2011 Mar;24(3):645-55. doi: 10.1111/j.1420-9101.2010.02203.x. Epub 2011 Jan 24. J Evol Biol. 2011. PMID: 21261769
-
A resource allocation model describing consequences of artificial selection under metabolic stress.
van der Waaij EH. van der Waaij EH. J Anim Sci. 2004 Apr;82(4):973-81. doi: 10.2527/2004.824973x. J Anim Sci. 2004. PMID: 15080316
-
The evolution of growth trajectories: what limits growth rate?
Dmitriew CM. Dmitriew CM. Biol Rev Camb Philos Soc. 2011 Feb;86(1):97-116. doi: 10.1111/j.1469-185X.2010.00136.x. Biol Rev Camb Philos Soc. 2011. PMID: 20394607 Review.
-
Mechanisms linking energy balance and reproduction: impact of prenatal environment.
Rhinehart EM. Rhinehart EM. Horm Mol Biol Clin Investig. 2016 Jan;25(1):29-43. doi: 10.1515/hmbci-2016-0004. Horm Mol Biol Clin Investig. 2016. PMID: 26943613 Review.
Cited by
-
The private life of malaria parasites: Strategies for sexual reproduction.
Schneider P, Reece SE. Schneider P, et al. Mol Biochem Parasitol. 2021 Jul;244:111375. doi: 10.1016/j.molbiopara.2021.111375. Epub 2021 May 20. Mol Biochem Parasitol. 2021. PMID: 34023299 Free PMC article. Review.
-
Competition and the evolution of reproductive restraint in malaria parasites.
Pollitt LC, Mideo N, Drew DR, Schneider P, Colegrave N, Reece SE. Pollitt LC, et al. Am Nat. 2011 Mar;177(3):358-67. doi: 10.1086/658175. Am Nat. 2011. PMID: 21460544 Free PMC article.
-
The good, the bad and the recovery in an assisted migration.
Green BS, Gardner C, Linnane A, Hawthorne PJ. Green BS, et al. PLoS One. 2010 Nov 30;5(11):e14160. doi: 10.1371/journal.pone.0014160. PLoS One. 2010. PMID: 21151965 Free PMC article.
-
Ejsmond A, Ejsmond MJ. Ejsmond A, et al. Ecol Evol. 2022 Dec 21;12(12):e9637. doi: 10.1002/ece3.9637. eCollection 2022 Dec. Ecol Evol. 2022. PMID: 36568869 Free PMC article.
-
Gutiérrez R, Córdova-Lepe F, Moreno-Gómez FN, Velásquez NA. Gutiérrez R, et al. Sci Rep. 2020 Dec 8;10(1):21401. doi: 10.1038/s41598-020-77326-1. Sci Rep. 2020. PMID: 33293662 Free PMC article.
References
-
- Agrawal AA. Phenotypic plasticity in the interactions and evolution of species. Science. 2001;294:321–326. - PubMed
-
- Benton TG, Grant A. Optimal reproductive effort in stochastic, density-dependent environments. Evolution. 1999;53:677–688. - PubMed
-
- Brown GP, Weatherhead PJ. Sexual abstinence and the cost of reproduction in adult male water snakes, Nerodia sipedon. Oikos. 2004;104:269–276.
-
- Bull JJ, Shine R. Iteroparous animals that skip opportunities for reproduction. American Naturalist. 1979;114:296–303.
-
- Clark CW, Harvell CD. Inducible defenses and the allocation of resources: a minimal model. American Naturalist. 1992;139:521–539.
Publication types
MeSH terms
LinkOut - more resources
Full Text Sources