Activation of the PI3 kinase pathway by retinoic acid mediates sodium/iodide symporter induction and iodide transport in MCF-7 breast cancer cells - PubMed
- ️Thu Jan 01 2009
Activation of the PI3 kinase pathway by retinoic acid mediates sodium/iodide symporter induction and iodide transport in MCF-7 breast cancer cells
Emi Ohashi et al. Cancer Res. 2009.
Abstract
Iodide uptake in the thyroid and breast is mediated by the sodium/iodide symporter (NIS). NIS activation is used for radioiodide imaging and therapeutic ablation of thyroid carcinoma. NIS is expressed in >70% of breast cancers but at a level insufficient for radioiodine treatment. All-trans retinoic acid (tRA) induces NIS gene expression and functional iodide uptake in human breast cancer cell lines and mouse breast cancer models. tRA usually regulates gene expression by direct interaction of RA receptor (RAR) with a target gene, but it can also act through nongenomic pathways. We report a direct influence of tRA treatment on the phosphoinositide 3-kinase (PI3K) signal transduction pathway that mediates tRA-induced NIS expression in MCF-7 breast cancer cells. MCF-7 cells express all three RAR isoforms, alpha, beta, and gamma, and RXRalpha. We previously identified RARbeta and RXRalpha as important for NIS induction by tRA. Treatment with LY294002, the PI3K inhibitor, or p85alpha knockdown with siRNA abolished tRA-induced NIS expression. Immunoprecipitation experiments and glutathione S-transferase pull-down assay showed a direct interaction between RARbeta2, RXRalpha, and p85alpha. RA also induced rapid activation of Akt in MCF-7 cells. Treatment with an Akt inhibitor or Akt knockdown with siRNA reduced NIS expression. These findings indicate that RA induction of NIS in MCF-7 cells is mediated by rapid activation of the PI3K pathway and involves direct interaction with RAR and retinoid X receptor. Defining these mechanisms should lead to methods to further enhance NIS expression, as well as retinoid targets that influence growth and differentiation of breast cancer.
Figures
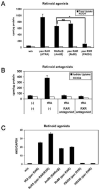
Influence of retinoids on NIS mRNA expression and iodide uptake in MCF-7 cells. A. Response to treatment with natural and synthetic RAR/RXR ligands. MCF7 cells were incubated with each ligand at a concentration of 1 μM for 24 h and iodide uptake was measured. Perchlorate (ClO4) (30 μM) is a specific inhibitor of NIS function, and was used to determine NIS-independent 125I-transport. *, statistical significane at a P value of <0.05; NS, not significant. B. pan-RAR and -RXR antagonists block the iodide uptake induced by tRA and 9cRA. MCF7 cells were incubated with a combination of RA (0.1 μM) and an antagonist (5 μM) for 24 h and iodide uptake was measured. C. Effect of RAR/RXR ligands on NIS mRNA expression. MCF7 cells were incubated with each ligand at a concentration of 1 μM for 12 h, and RT-qPCR of NIS and GAPDH mRNA was performed. The average ratio of NIS/GAPDH in untreated cells was set as 1.
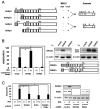
RARβ2 and RXRα are required for NIS induction by tRA. A. Schematic representation of RARβ variants expressed in MCF-7 cells. B. Stable transfection (Tx) of RARβ2 and β4 expression vectors. RT-qPCR for NIS and GAPDH mRNA was performed with transfected cells treated with or without tRA (1 μM) for 12 h (left panel). The average ratio of NIS/GAPDH in untreated cells was set as 1. *, statistical significance from empty vector transfected cells (P <0.01). RT-PCR confirming the expression of RARβ variants in stable transfected cells (right panel). Lower right panel shows the position of PCR primers used in B. RARβ2 primers detect RARβ2 only (left arrow below RARβ2), while RARβ primers detect both β2 and β4 (right arrow below both RARβ2 and β4). C. MCF-7 cells transfected with siRNAs against RARβ2 and/or RXRα were treated with 1 μM tRA for 12 h and RT-qPCR for NIS and GAPDH was performed (left panel). The average ratio of NIS/GAPDH in untreated cells was set as 1. Control siRNA; non-targeting siRNAs; *, statistical significance compared to control (P <0.01). Efficiency of the knockdown is shown by RT-PCR of RARβ2, RXRα and β-actin mRNA (right panel).
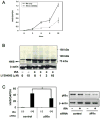
The PI3K inhibitor, LY294002, blocks NIS induction by tRA in MCF-7 cells in a time- and dose-dependent manner. A. LY294002 blocks tRA-induction of NIS mRNA. Cells were incubated with tRA (1 μM) with/without 10 μM LY294002 for indicated time and RT-qPCR for NIS and GAPDH was performed. B. LY294002 blocks tRA-induction of NIS protein expression in a dose-dependent fashion. Cells were incubated with 1 μM tRA with and without the indicated concentration of LY294002 for 24 h. The postnuclear membrane fraction was prepared with the tRA and LY294002 treated cells. Protein (30 μg) was applied to each lane for Western blot analysis, position of NIS protein shown by arrow. The same blot was stripped and incubated with β-actin antibody. C. MCF-7 cells transfected with p85α siRNA were treated with/without tRA (1 μM) for 12 h and RT-qPCR for NIS and GAPDH was performed (left panel). The average ratio of NIS/GAPDH in untreated cells was set as 1. Control siRNA; non-targeting siRNAs; *, statistical significance compared to control (P <0.01). Efficiency of the knockdown is shown by RT-PCR of p85α and β-actin (right panel).
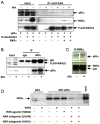
RARβ2 and RXRα interact with p85α. A and B. COS-7 cells were grown in 100 mm culture dishes and transfected with the indicated expression vector (total 4.5 μg). Twenty-four hours after transfection, cells were serum starved and treated with 1 μM tRA for 30 min. Whole-cell extracts (3 mg) were immunoprecipitated with either anti-FLAG antibody (A) or anti-p85α antibody (B) and then immunoblotted with the indicated antibodies. Immunoprecipitation with a goat anti-rabbit IgG was used to show the nonspecific binding, and the samples without immunoprecipitation (input) were used to confirm the expression of each protein. C. Increased association of RXRα with p85α by treatment with 1 μM tRA in co-immunoprecipitation study. Cells were serum starved and treated with 1 μM tRA for 30 min. Whole-cell extracts (3 mg) were immunoprecipitated with anti-p85α antibody and then immunoblotted with RXRα and p85α. D. Effect of natural and synthetic retinoids on human recombinant RXRα and p85α association in GST-p85α pull-down assay. RXRα was incubated with GST-p85α fusion protein in the presence of 1 μM of an RXR ligand (9cRA and HX630), a pan-RAR antagonist (LE540) or a pan-RXR antagonist (HX531).
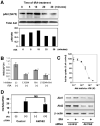
Rapid activation of Akt is involved in tRA-induction of NIS. A. Time-dependent effects of treatment with 1 μM tRA on Ser-473 phosphorylation of Akt. MCF-7 cells were serum-starved and treated with 1 μM tRA for the indicated time period. Whole cell lysates (20 μg) were used for Western blot analysis. Band density was measured by ImageJ software. B. Akt inhibitor VIII or PI3 kinase inhibitor LY294002 reduces RA-induction of NIS. MCF-7 cells were incubated with 1 μM tRA and the addition of Akt and/or PI3kinase inhibitor (10 μM) for 12 h, and then RT-qPCR for NIS and GAPDH were performed. The average ratio of NIS/GAPDH in untreated cells was set as 1. *, statistical significance compared to RA-treated cells (P <0.01). C. Influence of the Akt inhibitor VIII at increasing concentration on iodide uptake in MCF7 cells (IC50 4.5 μM). D. MCF-7 cells transfected with siRNAs targeted for Akt1 and Akt2 were treated with/without 1 μM tRA for 12 h and RT-qPCR for NIS and GAPDH was performed (right panel). The average ratio of NIS/GAPDH in untreated cells was set as 1. Control siRNA, non-targeting siRNAs; NS, not significant. Efficiency of the knockdown is shown by RT-PCR of Akt 1/Akt2 and β-actin (right panel).
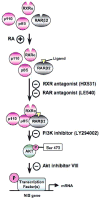
Model for the mechanisms of retinoid induction of NIS expression in MCF-7 cells. Factors that stimulate expression are shown with a “+” and those that inhibit are shown by a “-”.
Similar articles
-
Kogai T, Ohashi E, Jacobs MS, Sajid-Crockett S, Fisher ML, Kanamoto Y, Brent GA. Kogai T, et al. J Clin Endocrinol Metab. 2008 May;93(5):1884-92. doi: 10.1210/jc.2007-1627. Epub 2008 Mar 4. J Clin Endocrinol Metab. 2008. PMID: 18319322 Free PMC article.
-
Kogai T, Kanamoto Y, Li AI, Che LH, Ohashi E, Taki K, Chandraratna RA, Saito T, Brent GA. Kogai T, et al. Endocrinology. 2005 Jul;146(7):3059-69. doi: 10.1210/en.2004-1334. Epub 2005 Apr 7. Endocrinology. 2005. PMID: 15817668
-
Kogai T, Schultz JJ, Johnson LS, Huang M, Brent GA. Kogai T, et al. Proc Natl Acad Sci U S A. 2000 Jul 18;97(15):8519-24. doi: 10.1073/pnas.140217197. Proc Natl Acad Sci U S A. 2000. PMID: 10890895 Free PMC article.
-
Enhancement of sodium/iodide symporter expression in thyroid and breast cancer.
Kogai T, Taki K, Brent GA. Kogai T, et al. Endocr Relat Cancer. 2006 Sep;13(3):797-826. doi: 10.1677/erc.1.01143. Endocr Relat Cancer. 2006. PMID: 16954431 Review.
-
The sodium iodide symporter (NIS): regulation and approaches to targeting for cancer therapeutics.
Kogai T, Brent GA. Kogai T, et al. Pharmacol Ther. 2012 Sep;135(3):355-70. doi: 10.1016/j.pharmthera.2012.06.007. Epub 2012 Jun 29. Pharmacol Ther. 2012. PMID: 22750642 Free PMC article. Review.
Cited by
-
Guerrieri F, Piconese S, Lacoste C, Schinzari V, Testoni B, Valogne Y, Gerbal-Chaloin S, Samuel D, Bréchot C, Faivre J, Levrero M. Guerrieri F, et al. Cell Death Dis. 2013 Sep 19;4(9):e807. doi: 10.1038/cddis.2013.302. Cell Death Dis. 2013. PMID: 24052075 Free PMC article.
-
Ryan J, Curran CE, Hennessy E, Newell J, Morris JC, Kerin MJ, Dwyer RM. Ryan J, et al. PLoS One. 2011 Jan 19;6(1):e16023. doi: 10.1371/journal.pone.0016023. PLoS One. 2011. PMID: 21283523 Free PMC article.
-
Breast cancer brain metastases express the sodium iodide symporter.
Renier C, Vogel H, Offor O, Yao C, Wapnir I. Renier C, et al. J Neurooncol. 2010 Feb;96(3):331-6. doi: 10.1007/s11060-009-9971-8. Epub 2009 Jul 19. J Neurooncol. 2010. PMID: 19618116
-
microRNA-339-5p modulates Na+/I- symporter-mediated radioiodide uptake.
Lakshmanan A, Wojcicka A, Kotlarek M, Zhang X, Jazdzewski K, Jhiang SM. Lakshmanan A, et al. Endocr Relat Cancer. 2015 Feb;22(1):11-21. doi: 10.1530/ERC-14-0439. Epub 2014 Nov 17. Endocr Relat Cancer. 2015. PMID: 25404690 Free PMC article.
-
Agudo M, Yip P, Davies M, Bradbury E, Doherty P, McMahon S, Maden M, Corcoran JP. Agudo M, et al. Neurobiol Dis. 2010 Jan;37(1):147-55. doi: 10.1016/j.nbd.2009.09.018. Epub 2009 Oct 2. Neurobiol Dis. 2010. PMID: 19800972 Free PMC article.
References
-
- Kogai T, Kanamoto Y, Li AI, et al. Differential regulation of sodium/iodide symporter gene expression by nuclear receptor ligands in MCF-7 breast cancer cells. Endocrinology. 2005;146:3059–69. - PubMed
-
- Kogai T, Taki K, Brent GA. Enhancement of sodium/iodide symporter expression in thyroid and breast cancer. Endocr Relat Cancer. 2006;13:797–826. - PubMed
-
- Cho JY, Leveille R, Kao R, et al. Hormonal regulation of radioiodide uptake activity and Na+/I-symporter expression in mammary glands. J Clin Endocrinol Metab. 2000;85:2936–43. - PubMed
-
- Tazebay UH, Wapnir IL, Levy O, et al. The mammary gland iodide transporter is expressed during lactation and in breast cancer. Nat Med. 2000;6:871–8. - PubMed
-
- Wapnir IL, van de Rijn M, Nowels K, et al. Immunohistochemical profile of the sodium/iodide symporter in thyroid, breast, and other carcinomas using high density tissue microarrays and conventional sections. J Clin Endocrinol Metab. 2003;88:1880–8. - PubMed
Publication types
MeSH terms
Substances
LinkOut - more resources
Full Text Sources
Other Literature Sources
Medical