From rapid place learning to behavioral performance: a key role for the intermediate hippocampus - PubMed
- ️Thu Jan 01 2009
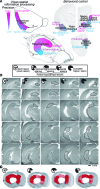
(A) Schematic summary of main functional connectivity of the hippocampus, which is shown in a rat brain with midline (ml) and rhinal fissure (rf) indicated for orientation (center, drawing adapted from Figure 1A of [32]). Hippocampal connectivity is topographically organized along a septotemporal (magenta-blue) gradient. Connections decline either toward the septal pole (close to the septum) or toward the temporal pole (close to the amygdala), so that they are largely restricted to approximately one- to two-thirds starting from either pole. Thus, a differentiation into three partly overlapping domains emerges with distinct sets of connectivity: a septal and temporal region, and, between them, an intermediate region. The septotemporal topography of connectivity to the entorhinal cortex [33,127,128], the main link to visuospatial processing (top left), and to medial prefrontal cortices [–131] and subcortical sites (mediodorsal striatum and nucleus accumbens [60,132,133], the amygdala [30,118], lateral septum, and hypothalamus [31,134]), which link the hippocampus to behavioral control (i.e., emotional, motivational, sensorimotor, and executive functions) (right), is indicated by magenta-to-blue coloration corresponding to different septotemporal levels of the hippocampus. Note that, within the entorhinal cortex, connectivity to neocortical sites conveying highly processed visuospatial information [135] and the precision at which entorhinal grid cells represent visuospatial information [15,34] decline from dorsolateral to ventromedial parts (magenta to blue). Reciprocal connections with the entorhinal cortex and projections to nucleus accumbens and lateral septum are related to the entire septotemporal hippocampal axis, whereas the projections to the medial prefrontal cortex (prelimbic–infralimbic cortex) and the reciprocal connectivity with the amygdala are restricted to the intermediate and temporal hippocampus. Direct projections to hypothalamic nuclei and mediodorsal striatum largely originate from the temporal portions of the hippocampus (including temporal aspects of the intermediate region). Overall, the septal to intermediate hippocampus, via connections to the dorsolateral portions of the entorhinal cortex, are functionally associated with precise visuospatial processing underlying rapid accurate place encoding; the temporal to intermediate hippocampus, via connections to medial prefrontal cortex and subcortical sites, are functionally linked to behavioral control. A convergence of links to precise visuospatial processing and to behavioral control is essentially restricted to the intermediate hippocampus. (B) Cresyl-violet–stained coronal sections through the hippocampus (hippoc.) of exemplar brains from the five groups in experiments 1 and 2 (from left to right): sham lesion, i.e., an intact hippocampus; partial lesions, sparing continuous chunks of approximately 40% of total hippocampal volume in the intermediate region, at the temporal pole, or at the septal pole; complete hippocampal lesion, i.e., virtually no intact hippocampus (sections are arranged from anterior to posterior, with the most anterior section at the top; lesions were bilateral, but to save space, only one hemisphere is used for illustration). In the hippocampus drawings that are used to indicate the different lesion groups (in this and the following figures), white represents intact tissue, and black indicates lesioned tissue. Lesions occasionally resulted in complete removal of the targeted tissue, mainly in the complete hippocampal lesion group, but more commonly in degenerated tissue without intact neurons (outlined by stippled line). (C) Three-dimensional reconstruction of bilateral hippocampal volume prepared from the coronal sections of the brains with a sham-lesion and with the three different partial hippocampal lesions. Intact hippocampal tissue is shown in dark red. In the brains with partial hippocampal lesions, the volume of the intact (control) hippocampus is indicated in light red for comparison. Apart from the hippocampus, the brain silhouette (gray), with the midline (ml) and the rhinal fissure (rf), is shown for orientation; the silhouette is rendered transparent where it would otherwise cover the view of the hippocampus. The residual hippocampal volumes in the exemplar brains with partial hippocampal lesions shown in (B) and (C) were 41% in the intermediate region, 49% at the temporal pole, and 42% at the septal pole, respectively. In the exemplar brain with intended sparing at the septal pole, there was also some unintended sparing of a small volume (5%) at the temporal pole; this temporal sparing appears relatively large in the depicted view of the three-dimensional reconstruction, but an all-around view of the reconstruction clearly shows that it is only a very small piece of tissue. See Videos S1–S4 for an all-around view of the reconstructions.
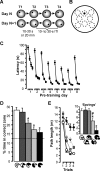
(A) Rats had four daily trials (T1–T4) in the watermaze. The location of the hidden escape platform (black dot) was constant within a day, but the platform was moved to a novel location at the beginning of each day. Thus, rapid place learning during T1 of each day enabled efficient performance during T2 and the subsequent trials. The retention delay between T1 and T2 was 10–30 s or 20 min, whereas all other intertrial intervals (ITI) within a day were 10–30 s. T2 was occasionally run as probe with the platform not coming up until after 60 s, during which time the search preference for the area containing the platform location could be measured. (B) Zone analysis of search preference on probes: eight 40-cm-diameter zones (stippled circles) were defined within the 2-m diameter of the watermaze, including the correct zone, which was concentric with the location of the platform (12-cm diameter; black dot) on T1 of the day. The zones were nonoverlapping, evenly spaced, and symmetrically arranged. The time rats spent in the different zones during the 60-s probe trial was measured, and the percentage of time spent in the correct zone was calculated as: (time in the correct zone/time in all eight zones) × 100%. By chance, i.e., during random swimming, this value should be 12.5%, whereas higher values indicate a search preference for the correct zone based on one-trial place learning. (C) To illustrate the task principle, latencies (mean ± SEM) to reach the platform are plotted for T1–T4 across the eight pretraining days preceding the lesion surgery. Rats (n = 89) typically showed high latencies on T1, reflecting searching for the novel platform location, and substantially reduced latencies during subsequent trials, reflecting more efficient navigation based on the use of information learned during the preceding trials (main effect of four daily trials, F 3,252 = 419.8, p < 0.0001). Especially striking were the latency reductions (savings) from T1 to T2 (t 88 = 19.05, p < 0.0001), demonstrating one-trial learning. Across days, mainly the first 4 d, there was an overall decrease in latencies (main effect of days, F 7,588 = 84.9, p < 0.0001) and improved latency reductions between trials (interaction days × trials: F 21,1764 = 5.09, p < 0.0001), indicating learning of the general task demands (swimming, moving away from pool wall, win-stay rule). Data were collapsed for the two different T1–T2 delays (10–30s or 20 min), which were used equally often and for half of the rats each on every day. On days 4 and 8, T2 was run as probe. (D and E) Postsurgical performance: (D) percentage time (%time) in correct zone (mean ± SEM) on probes and (E) path lengths (mean ± SEM) to reach the platform on T1–T4, as well as path-length reductions from T1 to T2 (savings, inset graph) in sham-lesioned rats, in rats with approximately 40% of residual hippocampal volume in the intermediate, temporal, or septal hippocampus, and in rats with complete hippocampal lesions (compare Figure 1B and 1C). Group differences: an asterisk (*) indicates different from groups without asterisk (p < 0.025); a number sign (#) indicates different from group with complete hippocampal lesions (p < 0.025); no additional significant differences (p > 0.05). All groups showed higher than chance (indicated by stippled line) search preference and significant path-length reductions from trial 1 to 2 (t > 4.4, p < 0.04), except for the one with complete hippocampal lesions (t < 3.0, p > 0.16).
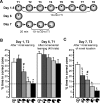
(A) Rats were trained to one constant platform location on eight daily trials (T1–T8) for 5 d, with T2 and T6 of each day run as probes to closely monitor performance improvements. The intertrial interval (ITI) was 10 min. On day 6, one additional probe trial was run to assess performance based on incremental place learning across the five preceding days unconfounded by within-day learning. On day 7, rats were retested on the rapid place-learning task, with four trials to a novel location and T2 run as probe. The retention delay between T1 and T2 was 20 min, and the other ITIs were 10–30 s. (B) Search preference for the correct zone (mean ± SEM) after one-trial place-learning (day 1, T2) and after 40 trials of slow, incremental learning (day 6, T1) in the different lesion groups (compare Figure 1B and 1C). ANOVA indicated significant group differences only on day 1, T2 (see main text), and results of the post hoc comparisons are indicated: an asterisk (*) indicates different from groups without an asterisk (p < 0.015); a number sign (#) indicates different from the group with the hippocampal residual at the septal pole and the one with complete hippocampal lesions (p < 0.04); no additional significant differences (p > 0.4). On day 1, T2, search preference for the correct zone was above chance (stippled line) in rats with sham-lesions and with hippocampal residuals in the intermediate region or at the temporal pole (t > 3.1, p < 0.01), but not in rats with hippocampal residuals at the septal pole or with complete hippocampal lesions (t < 1.5, p > 0.19). In contrast, all groups showed a significant preference for the correct zone on day 6, T1 (t > 4.8, p < 0.0005). (C) When retested for performance based on one-trial place learning on day 7, T2, there were marked group differences in search preference for the correct zone (mean ± SEM): an asterisk (*) indicates different from sham-operated group (p < 0.025); a number sign (#) indicates different from group with complete hippocampal lesions (p < 0.025); a section symbol (§) indicates different from group with complete hippocampal lesions and group with only the septal pole spared (p < 0.01); no additional significant differences (p > 0.09). Sham-operated rats and those with a residual intermediate hippocampus showed a significant preference for the correct zone (t > 3.9, p < 0.002), whereas rats with hippocampal residuals at septal or temporal pole did not differ from chance (t < 1.2, p > 0.26), and rats with complete hippocampal lesions spent even less time in the correct zone than expected by chance (t 13 = 4.93, p < 0.0004).
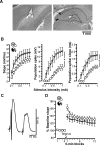
(A) Electrolytic lesions (white arrowheads) marking the stimulation site in the perforant path (bipolar electrode, left) and the recording site in the ipsilateral dentate gyrus in cresyl-violet–stained coronal sections. Photos are from a brain with partial hippocampal lesion sparing the septal pole (35% of hippocampal volume), and damage to CA3 (black arrowheads) is visible at the level of the recording site. (B) Input–output curves recorded from rats with sham lesions or with partial hippocampal lesions sparing residuals at the septal pole: different measures of the evoked field response (mean ± SEM) are plotted as function of the stimulus intensity (0.1–1 mA). An asterisk (*) indicates significant main effect of group (p < 0.03). (C) Paired-pulse inhibition in a septal hippocampal residual: field responses evoked by paired stimulation (0.5 mA, 20 ms apart; average of 15 samples). Note the large population spike in the first response and its virtual absence in the second response. The residual hippocampal volume in this case was 27%. (D) Brief tetanic stimulation induces similar LTP of the evoked response in rats with sham lesions and with partial hippocampal lesions sparing residuals at the septal pole. The evoked response is expressed as percentage baseline slope (mean ± SEM).
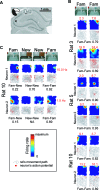
(A) Cresyl-violet–stained coronal section showing the tracks of the four tetrode bundles (circled by stippled line; individual tracks indicated by black dots) used to record place-related activity from the CA1 region of the septal hippocampus; tracks were marked by passing an electric current through the tetrodes after completion of the recordings. The section is from rat 10, which had a hippocampal residual of 26% at the septal pole. A slight disruption of the CA3 layer is visible (black arrowhead), indicating the beginning of the lesion to intermediate and temporal hippocampus. (B) CA1 cells in hippocampal residuals at the septal pole show accurate and stable place-related firing in a familiar environment (Fam): Firing fields of complex-spike cells recorded from three rats (3, 5, and 10) with hippocampal residuals at the septal pole during two successive trials in the familiar environment. The color-coded firing-rate maps and, below, the location of spikes (red) on the rat's trajectory (gray) are shown for the two cells of each rat with the highest spatial-information score. The peak firing rate for each trial is indicated in red numbers above and the correlation between the firing-rate maps for the two successive trials is shown in black below. Note that neurons often changed their peak firing rate between trials, but that the firing positions remained stable. See Figure S5A and S5B for additional cells recorded from the rats with hippocampal residuals at the septal pole and for cells recorded from a control group with intact hippocampus. (C) CA1 cells in hippocampal residuals at the septal pole rapidly change their firing pattern in a new environment. Firing fields of two complex-spike cells recorded from one rat with hippocampal residuals at the septal pole (rat 10; the two neurons are not identical with the two neurons whose firing patterns are shown in [B]) during a succession of trials in the familiar (Fam) and a new (New) environment. All firing-rate maps are scaled to the same maximum firing rate, equal to the maximum of all four trials and indicated in red to the right; correlations between the firing-rate maps for different trials are indicated in black below. Note that neuron 1 forms a new place field in the new environment, whereas neuron 2 becomes nearly silent. See Figure S6 for additional cells from rat 10 and from two control rats with intact hippocampus.
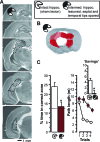
(A) Cresyl-violet–stained coronal sections through the hippocampus of one exemplar brain with lesion to the intermediate hippocampus (arranged from most anterior, top left, to most posterior, bottom right; degenerated tissue without intact neurons outlined by stippled line). (B) Three-dimensional reconstruction of bilateral hippocampal volume in the same brain (compare Figure 1C for explanation and abbreviations). The residual hippocampal volume in the exemplar brain was 43%: 21% at the temporal and 22% at the septal tip. See Video S5 for an all-around view. (C) Performance on the rapid place-learning task in the watermaze (compare Figure 2A), as reflected by percentage time (% time) in correct zone on probes and path lengths on T1–T4, as well as path-length reductions from T1 to T2 (savings, inset graph) (all data: mean ± SEM), in rats with lesions to the intermediate hippocampus and in sham-operated rats. An asterisk (*) indicates significant group differences (p < 0.005). Sham-operated rats showed higher than chance search preference and a significant path-length reduction from trial 1 to 2 (t 11 > 5.23, p < 0.0003), but rats with lesions to the intermediate hippocampus did not (t 10 < 0.41).