Epilepsy, ataxia, sensorineural deafness, tubulopathy, and KCNJ10 mutations - PubMed
- ️Thu Jan 01 2009
. 2009 May 7;360(19):1960-70.
doi: 10.1056/NEJMoa0810276.
Sally Feather, Horia C Stanescu, Sascha Bandulik, Anselm A Zdebik, Markus Reichold, Jonathan Tobin, Evelyn Lieberer, Christina Sterner, Guida Landoure, Ruchi Arora, Tony Sirimanna, Dorothy Thompson, J Helen Cross, William van't Hoff, Omar Al Masri, Kjell Tullus, Stella Yeung, Yair Anikster, Enriko Klootwijk, Mike Hubank, Michael J Dillon, Dirk Heitzmann, Mauricio Arcos-Burgos, Mark A Knepper, Angus Dobbie, William A Gahl, Richard Warth, Eamonn Sheridan, Robert Kleta
Affiliations
- PMID: 19420365
- PMCID: PMC3398803
- DOI: 10.1056/NEJMoa0810276
Epilepsy, ataxia, sensorineural deafness, tubulopathy, and KCNJ10 mutations
Detlef Bockenhauer et al. N Engl J Med. 2009.
Abstract
Background: Five children from two consanguineous families presented with epilepsy beginning in infancy and severe ataxia, moderate sensorineural deafness, and a renal salt-losing tubulopathy with normotensive hypokalemic metabolic alkalosis. We investigated the genetic basis of this autosomal recessive disease, which we call the EAST syndrome (the presence of epilepsy, ataxia, sensorineural deafness, and tubulopathy).
Methods: Whole-genome linkage analysis was performed in the four affected children in one of the families. Newly identified mutations in a potassium-channel gene were evaluated with the use of a heterologous expression system. Protein expression and function were further investigated in genetically modified mice.
Results: Linkage analysis identified a single significant locus on chromosome 1q23.2 with a lod score of 4.98. This region contained the KCNJ10 gene, which encodes a potassium channel expressed in the brain, inner ear, and kidney. Sequencing of this candidate gene revealed homozygous missense mutations in affected persons in both families. These mutations, when expressed heterologously in xenopus oocytes, caused significant and specific decreases in potassium currents. Mice with Kcnj10 deletions became dehydrated, with definitive evidence of renal salt wasting.
Conclusions: Mutations in KCNJ10 cause a specific disorder, consisting of epilepsy, ataxia, sensorineural deafness, and tubulopathy. Our findings indicate that KCNJ10 plays a major role in renal salt handling and, hence, possibly also in blood-pressure maintenance and its regulation.
2009 Massachusetts Medical Society
Conflict of interest statement
Dr. Sheridan reports receiving grant support from the Well-being of Women. No other potential conflict of interest relevant to this article was reported.
Figures
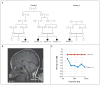
The pedigree of Family 1 (Panel A) shows four affected members, and that of Family 2 one affected member. Squares indicate male family members and circles female family members; filled squares and circles indicate that the patients are affected. Double lines between parents indicate that the parents are related. An MRI scan of the brain obtained from Patient 1-1 at 9 years of age (Panel B) shows normal cerebral and cerebellar structures. An audiogram of the left ear in the same patient at 14 years of age (Panel C) shows moderate hearing loss, which is more pronounced at higher frequencies. A hearing deficit of up to 20 dB is considered normal for frequencies from 250 to 8000 Hz.
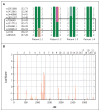
A haplotype reconstruction (Panel A) for the locus on chromosome 1 shows identical alleles (indicated by the same color) in the linked region in all affected patients. The numbers (i.e., 1 or 2) next to the alleles indicate the respective status of the single-nucleotide polymorphisms used for genotyping. Recombinations in Patients 1-1 and 1-2 define this region. The parametric multipoint linkage analysis of the whole genome for Family 1 (Panel B) has a single significant peak, with a maximum lod score of almost 5 on chromosome 1. Genetic distance (in centimorgans) and individual chromosomes (1 to 22) are indicated on the lower and upper x axes, respectively.
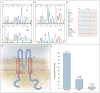
Panel A shows sequence chromatograms for a wild-type (WT) control and Patient 1-1. The homozygous missense mutation c.194G C (CGC CCC; p.R65P) is present in the patient’s chromatogram (arrow). Panel B shows sequence chromatograms for a wild-type control and Patient 2-1. The homozygous missense mutation c.229 G C (GGC CGC; p.G77R) is present in the patient’s chromatogram (arrow). Panel C shows a protein-alignment (homology) plot of the first transmembrane region of KCNJ10 in 21 vertebrate species, with complete conservation of R65 and G77 (arrows). A model based on the crystal structure of a bacterial homologue of KCNJ10, (i.e., kcsa), in Panel D, shows the localization of mutations within the first transmembrane region. When heterologous expression of wild-type KNCJ10 and mutants (R65P and G77R) in xenopus oocytes was measured with the use of a two-electrode voltage clamp, a significant decrease of specific currents in mutant KCNJ10 was observed, as shown in Panel E. N denotes the number of experiments.
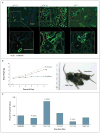
Serial sections of a kidney from a wild-type mouse (Panel A), stained for antibodies to the proteins NKCC2 (green, indicating luminal thick ascending limb), KCNJ10 (green), NCC (green, indicating luminal distal convoluted tubule), AQP2 (red, indicating principal cells of the luminal collecting duct), and calbindin (blue, indicating distal convoluted tubule and collecting duct) show staining for KCNJ10 basolaterally in the distal part of the nephron only. The glomerulus is indicated by an asterisk. Scale bars indicate 100 μm. Growth curves for wild-type mice, heterozygous (HE) mice, and homozygous (knockout) mice (based on the mean values for two animals of each type) show virtually no growth of the knockout mice (Panel B), which died after day 8. The photograph of two 6-day-old mice from the same litter, one knockout and one wild-type, shows the growth arrest in the knockout mouse. The bar graph (Panel C) shows the mean results of urinalysis for 7 knockout mice as compared with 29 wild-type and heterozygous mice, all at the age of 3 days. Significant results were obtained for creatinine concentration (Creat) (a decrease), sodium excretion (Na/Creat) (an increase), indicating renal salt wasting, and calcium excretion (Ca/Creat) (a decrease) in the knockout mice.
Comment in
-
Membrane physiology--bridging the gap between medical disciplines.
Bleich M. Bleich M. N Engl J Med. 2009 May 7;360(19):2012-4. doi: 10.1056/NEJMe0901812. N Engl J Med. 2009. PMID: 19420371 No abstract available.
-
The EAST syndrome and KCNJ10 mutations.
Shi M, Zhao G. Shi M, et al. N Engl J Med. 2009 Aug 6;361(6):630; author reply 630-1. doi: 10.1056/NEJMc091202. N Engl J Med. 2009. PMID: 19657131 No abstract available.
-
A K+ channel that channels neurology to nephrology.
Huang K. Huang K. Clin Genet. 2010 Mar;77(3):230-1. doi: 10.1111/j.1399-0004.2009.01322.x. Epub 2009 Nov 23. Clin Genet. 2010. PMID: 19968668 No abstract available.
Similar articles
-
Schlingmann KP, Renigunta A, Hoorn EJ, Forst AL, Renigunta V, Atanasov V, Mahendran S, Barakat TS, Gillion V, Godefroid N, Brooks AS, Lugtenberg D, Lake J, Debaix H, Rudin C, Knebelmann B, Tellier S, Rousset-Rouvière C, Viering D, de Baaij JHF, Weber S, Palygin O, Staruschenko A, Kleta R, Houillier P, Bockenhauer D, Devuyst O, Vargas-Poussou R, Warth R, Zdebik AA, Konrad M. Schlingmann KP, et al. J Am Soc Nephrol. 2021 Jun 1;32(6):1498-1512. doi: 10.1681/ASN.2020111587. Epub 2021 Apr 2. J Am Soc Nephrol. 2021. PMID: 33811157 Free PMC article.
-
Morin M, Forst AL, Pérez-Torre P, Jiménez-Escrig A, Barca-Tierno V, García-Galloway E, Warth R, Lopez-Sendón Moreno JL, Moreno-Pelayo MA. Morin M, et al. Neurogenetics. 2020 Apr;21(2):135-143. doi: 10.1007/s10048-020-00605-6. Epub 2020 Feb 15. Neurogenetics. 2020. PMID: 32062759
-
Reichold M, Zdebik AA, Lieberer E, Rapedius M, Schmidt K, Bandulik S, Sterner C, Tegtmeier I, Penton D, Baukrowitz T, Hulton SA, Witzgall R, Ben-Zeev B, Howie AJ, Kleta R, Bockenhauer D, Warth R. Reichold M, et al. Proc Natl Acad Sci U S A. 2010 Aug 10;107(32):14490-5. doi: 10.1073/pnas.1003072107. Epub 2010 Jul 22. Proc Natl Acad Sci U S A. 2010. PMID: 20651251 Free PMC article.
-
Bandulik S, Schmidt K, Bockenhauer D, Zdebik AA, Humberg E, Kleta R, Warth R, Reichold M. Bandulik S, et al. Pflugers Arch. 2011 Apr;461(4):423-35. doi: 10.1007/s00424-010-0915-0. Epub 2011 Jan 11. Pflugers Arch. 2011. PMID: 21221631 Review.
-
Epilepsy in patients with EAST syndrome caused by mutation in the KCNJ10.
Mir A, Chaudhary M, Alkhaldi H, Alhazmi R, Albaradie R, Housawi Y. Mir A, et al. Brain Dev. 2019 Sep;41(8):706-715. doi: 10.1016/j.braindev.2019.03.009. Epub 2019 Apr 2. Brain Dev. 2019. PMID: 30952461 Review.
Cited by
-
Polyamine transport by the polyspecific organic cation transporters OCT1, OCT2, and OCT3.
Sala-Rabanal M, Li DC, Dake GR, Kurata HT, Inyushin M, Skatchkov SN, Nichols CG. Sala-Rabanal M, et al. Mol Pharm. 2013 Apr 1;10(4):1450-8. doi: 10.1021/mp400024d. Epub 2013 Mar 19. Mol Pharm. 2013. PMID: 23458604 Free PMC article.
-
Kir4.1/Kir5.1 in the DCT plays a role in the regulation of renal K+ excretion.
Su XT, Ellison DH, Wang WH. Su XT, et al. Am J Physiol Renal Physiol. 2019 Mar 1;316(3):F582-F586. doi: 10.1152/ajprenal.00412.2018. Epub 2019 Jan 9. Am J Physiol Renal Physiol. 2019. PMID: 30623727 Free PMC article.
-
McClenahan SJ, Kent CN, Kharade SV, Isaeva E, Williams JC, Han C, Terker A, Gresham R 3rd, Lazarenko RM, Days EL, Romaine IM, Bauer JA, Boutaud O, Sulikowski GA, Harris R, Weaver CD, Staruschenko A, Lindsley CW, Denton JS. McClenahan SJ, et al. Mol Pharmacol. 2022 May;101(5):357-370. doi: 10.1124/molpharm.121.000464. Epub 2022 Mar 3. Mol Pharmacol. 2022. PMID: 35246480 Free PMC article.
-
Glioma epileptiform activity and progression are driven by IGSF3-mediated potassium dysregulation.
Curry RN, Aiba I, Meyer J, Lozzi B, Ko Y, McDonald MF, Rosenbaum A, Cervantes A, Huang-Hobbs E, Cocito C, Greenfield JP, Jalali A, Gavvala J, Mohila C, Serin Harmanci A, Noebels J, Rao G, Deneen B. Curry RN, et al. Neuron. 2023 Mar 1;111(5):682-695.e9. doi: 10.1016/j.neuron.2023.01.013. Epub 2023 Feb 13. Neuron. 2023. PMID: 36787748 Free PMC article.
-
Possible role for rare TRPM7 variants in patients with hypomagnesaemia with secondary hypocalcaemia.
Vargas-Poussou R, Claverie-Martin F, Prot-Bertoye C, Carotti V, van der Wijst J, Perdomo-Ramirez A, Fraga-Rodriguez GM, Hureaux M, Bos C, Latta F, Houillier P, Hoenderop JGJ, de Baaij JHF. Vargas-Poussou R, et al. Nephrol Dial Transplant. 2023 Feb 28;38(3):679-690. doi: 10.1093/ndt/gfac182. Nephrol Dial Transplant. 2023. PMID: 35561741 Free PMC article.
References
-
- Kleta R, Bockenhauer D. Bartter syndromes and other salt-losing tubulopathies. Nephron Physiol. 2006;104:p73–p80. - PubMed
-
- Simon DB, Nelson-Williams C, Bia MJ, et al. Gitelman’s variant of Bartter’s syndrome, inherited hypokalaemic alkalosis, is caused by mutations in the thiazide-sensitive Na-Cl cotransporter. Nat Genet. 1996;12:24–30. - PubMed
-
- Simon DB, Karet FE, Hamdan JM, DiPietro A, Sanjad SA, Lifton RP. Bartter’s syndrome, hypokalaemic alkalosis with hypercalciuria, is caused by mutations in the Na-K-2Cl cotransporter NKCC2. Nat Genet. 1996;13:183–8. - PubMed
-
- Simon DB, Karet FE, Rodriguez-Soriano J, et al. Genetic heterogeneity of Bartter’s syndrome revealed by mutations in the K+ channel, ROMK. Nat Genet. 1996;14:152–6. - PubMed
-
- Simon DB, Bindra RS, Mansfield TA, et al. Mutations in the chloride channel gene, CLCNKB, cause Bartter’s syndrome type III. Nat Genet. 1997;17:171–8. - PubMed
Publication types
MeSH terms
Substances
Grants and funding
LinkOut - more resources
Full Text Sources
Other Literature Sources
Medical
Molecular Biology Databases
Miscellaneous