Intermediate filaments: primary determinants of cell architecture and plasticity - PubMed
Review
. 2009 Jul;119(7):1772-83.
doi: 10.1172/JCI38214. Epub 2009 Jul 1.
Affiliations
- PMID: 19587452
- PMCID: PMC2701873
- DOI: 10.1172/JCI38214
Review
Intermediate filaments: primary determinants of cell architecture and plasticity
Harald Herrmann et al. J Clin Invest. 2009 Jul.
Abstract
Intermediate filaments (IFs) are major constituents of the cytoskeleton and nuclear boundary in animal cells. They are of prime importance for the functional organization of structural elements. Depending on the cell type, morphologically similar but biochemically distinct proteins form highly viscoelastic filament networks with multiple nanomechanical functions. Besides their primary role in cell plasticity and their established function as cellular stress absorbers, recently discovered gene defects have elucidated that structural alterations of IFs can affect their involvement both in signaling and in controlling gene regulatory networks. Here, we highlight the basic structural and functional properties of IFs and derive a concept of how mutations may affect cellular architecture and thereby tissue construction and physiology.
Figures
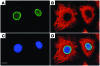
Indirect immunofluorescence staining with antibodies specific for (A) lamin A and (B) vimentin indicates that lamins are concentrated at the inner nuclear membrane within the nucleus, whereas vimentin forms a complex network within the cytoplasm that extends from the cell periphery immediately to the outer nuclear membrane. (C) DNA has been stained with the DNA-specific dye DAPI (blue). (D) Merged image of A–C. Scale bar: 10 μm.
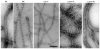
Negatively stained preparations of MTs, MFs, vimentin IFs (Cyt-IFs), lamin A filaments (Lamin-IFs), and paracrystalline lamin A fibers (Lamin-Pc), as visualized by transmission electron microscopy. Scale bar: 100 nm.
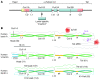
(A) Schematic representation of the structural organization of an IF molecule. Blue boxes represent segments; orange boxes represent IF-consensus motifs (CD); and the yellow box represents an α-helical pre-coil domain only found in vimentin-like IF proteins. L1, L12, and L2 are linker segments. As L2 is predicted to form an α-helical segment, coil 2A and linker L2 are designated as paired bundle (pb) in B. “Head” and “tail” represent non–α-helical domains. The stutter (st) indicates a discontinuity in the heptad repeat pattern of coil 2B (43). The blue box designated lamin-specific “insertion” highlights a position in which an additional α-helical segment is found in lamins and lower invertebrate cytoplasmic IF proteins. (B) Model of the dimeric structures exhibited by human lamin A, vimentin, and the epidermal pair K5/K14. Vimentin exhibits a pre-coil domain (PCD). Moreover, a special feature of nuclear lamins, as opposed to cytoplastic IF proteins, is that linker L1 in lamins is likely to be α-helical. The numbers in parentheses indicate the number of amino acids in the respective non–α-helical domains. NLS, nuclear localization signal. B is adapted from Nature reviews molecular cell biology (8).
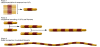
In phase 1, eight tetrameric subunits made from two antiparallel, half-staggered coiled-coil dimers associate laterally to form ULFs after initiation of assembly. Every single vimentin molecule is represented by one cylinder; coil 1 of each molecule is colored dark red, and coil 2 is colored yellow. In phase 2, ULFs and short filaments longitudinally anneal to other ULFs and filaments. In phase 3, after about ten minutes of assembly, filaments have radially compacted to a diameter of approximately 11 nm. Adapted with permission from Journal of biological chemistry (80).
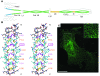
(A) Hypothetical model depicting that coil 1A may have a tendency to open up with the authentic tyrosine 117, shown in extreme conformation. (B) Side stereo view of the atomic structure of coil 1A from the vimentin mutant Y117L, exhibiting a bona fide coiled coil. Residues bounded by the first and the last knobs-into-hole interaction are colored in cyan. Residues at a positions are shown in yellow and those at d positions are shown in magenta. The N terminus is marked N, and the C terminus is marked C. The residue types and numbers of the core positions are indicated. (C) Transfection of mouse embryonic fibroblasts derived from vimentin-knockout mice (130) with the vimentin mutant Y117L, followed by indirect immunofluorescence microscopy with antibodies specific for human vimentin, revealing dot-like structures exclusively. Note the regular shaped particles that on occasion are lined up, possibly being situated on a fibrillar structure such as a MT (inset). Scale bar: 20 μm. A is reproduced with permission from EMBO journal (43). B and C are reproduced with permission from Journal of molecular biology (92).
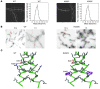
(A) Mass measurement of assembled wild-type desmin (WT) filaments in comparison with filaments formed by the mutant A360P. Nonstained, lightly fixed filaments were analyzed by scanning transmission electron microscopy and visualized in a dark field mode (first and third panels from the left). The rectangles indicate the areas used for the quantification of mass. One side of the square is 60-nm long. The molecular mass of individual filaments was determined along the various individual filaments and plotted as kDa/nm into a histogram (second and fourth panels from the left). The abscissa shows mass in kDa/nm; the ordinate shows the number of segments analyzed. (B) Filament assembly of wild-type desmin in comparison to that of the mutant R406W, as depicted by electron microscopy of negatively stained samples obtained at 10 seconds and 1 hour. Scale bar: 100 nm. (C) C-terminal region of the wild-type desmin dimer (left panel) compared with the R406W mutant (right panel). The mutated amino acid is shown in magenta. The change of arginine (R406) to tryptophan (W406) destroys the salt bridge formed with E401. The atomic model is based on the known crystal structure of the corresponding vimentin fragment. The figure is a composite of figures adapted with permission from Journal of molecular biology and Journal of structural biology (110, 131).
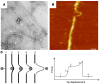
(C) Schematic description of the filament rupture. The gray disk depicts the atomic force microscopy tip tearing on a filament (black bar). The bottom diagram illustrates the force-displacement curve corresponding to the 5 phases shown in the upper panel. A and B were reproduced with permission from Journal of molecular biology (125). Scale bars: 100 nm.
Similar articles
-
[Intermediate-filament-associated diseases].
Paulin D, Diguet N, Xue Z, Li Z. Paulin D, et al. Biol Aujourdhui. 2011;205(3):139-46. doi: 10.1051/jbio/2011015. Epub 2011 Oct 11. Biol Aujourdhui. 2011. PMID: 21982403 Review. French.
-
Softness, strength and self-repair in intermediate filament networks.
Wagner OI, Rammensee S, Korde N, Wen Q, Leterrier JF, Janmey PA. Wagner OI, et al. Exp Cell Res. 2007 Jun 10;313(10):2228-35. doi: 10.1016/j.yexcr.2007.04.025. Epub 2007 Apr 27. Exp Cell Res. 2007. PMID: 17524395 Free PMC article. Review.
-
Intermediate filaments as signaling platforms.
Pallari HM, Eriksson JE. Pallari HM, et al. Sci STKE. 2006 Dec 19;2006(366):pe53. doi: 10.1126/stke.3662006pe53. Sci STKE. 2006. PMID: 17179489 Review.
-
Galou M, Gao J, Humbert J, Mericskay M, Li Z, Paulin D, Vicart P. Galou M, et al. Biol Cell. 1997 May;89(2):85-97. Biol Cell. 1997. PMID: 9351189 Review.
-
Intermediate filaments in smooth muscle.
Tang DD. Tang DD. Am J Physiol Cell Physiol. 2008 Apr;294(4):C869-78. doi: 10.1152/ajpcell.00154.2007. Epub 2008 Feb 6. Am J Physiol Cell Physiol. 2008. PMID: 18256275 Free PMC article. Review.
Cited by
-
Johnen N, Francart ME, Thelen N, Cloes M, Thiry M. Johnen N, et al. Histochem Cell Biol. 2012 Sep;138(3):477-88. doi: 10.1007/s00418-012-0969-5. Epub 2012 May 19. Histochem Cell Biol. 2012. PMID: 22610129
-
Intermediate Filaments: Structure and Assembly.
Herrmann H, Aebi U. Herrmann H, et al. Cold Spring Harb Perspect Biol. 2016 Nov 1;8(11):a018242. doi: 10.1101/cshperspect.a018242. Cold Spring Harb Perspect Biol. 2016. PMID: 27803112 Free PMC article. Review.
-
Karabinos A, Schünemann J, Parry DAD. Karabinos A, et al. J Mol Evol. 2019 Sep;87(7-8):221-230. doi: 10.1007/s00239-019-09904-5. Epub 2019 Aug 12. J Mol Evol. 2019. PMID: 31407015
-
Hnia K, Tronchère H, Tomczak KK, Amoasii L, Schultz P, Beggs AH, Payrastre B, Mandel JL, Laporte J. Hnia K, et al. J Clin Invest. 2011 Jan;121(1):70-85. doi: 10.1172/JCI44021. Epub 2010 Dec 6. J Clin Invest. 2011. PMID: 21135508 Free PMC article.
-
Xia B, Zhang H, Yang M, Du S, Wei J, Ding L. Xia B, et al. Int J Mol Sci. 2020 Apr 30;21(9):3164. doi: 10.3390/ijms21093164. Int J Mol Sci. 2020. PMID: 32365802 Free PMC article.
References
-
- Fuchs E., Weber K. Intermediate filaments: structure, dynamics, function, and disease. Annu. Rev. Biochem. 1994;63:345–382. - PubMed