Insights from genomic profiling of transcription factors - PubMed
Review
Insights from genomic profiling of transcription factors
Peggy J Farnham. Nat Rev Genet. 2009 Sep.
Abstract
A crucial question in the field of gene regulation is whether the location at which a transcription factor binds influences its effectiveness or the mechanism by which it regulates transcription. Comprehensive transcription factor binding maps are needed to address these issues, and genome-wide mapping is now possible thanks to the technological advances of ChIP-chip and ChIP-seq. This Review discusses how recent genomic profiling of transcription factors gives insight into how binding specificity is achieved and what features of chromatin influence the ability of transcription factors to interact with the genome. It also suggests future experiments that may further our understanding of the causes and consequences of transcription factor-genome interactions.
Figures
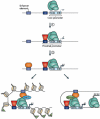
General transcription factors (green) bind to core promoter regions via recognition of common elements such as TATA boxes (TATA) and initiators (INR). However, these elements on their own provide very low levels of transcriptional activity due to unstable interactions of the general factors with the promoter region. Promoter activity can be increased by site-specific DNA binding factors (red) interacting with cis elements in the proximal promoter region and stabilizing the recruitment of the transcriptional machinery through direct interaction of the site-specific factor and the general factors (step 1). Promoter activity can be further stimulated to higher levels by site-specific factors (orange) binding to enhancers (step 2). The enhancer factors can stimulate transcription by (A) recruiting a histone-modifying enzyme (for example a histone acetyltransferease, HAT) to create a more favorable chromatin environment for transcription (acetylated histones, Ac) or (B) recruiting a kinase that can phosphorylate the C terminal domain of RNA polymerase II and stimulate elongation.
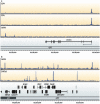
(A) Localization analysis reveals two classes of binding patterns for transcription factors. Shown is the analysis of binding sites identified using ChIP-seq for E2F4, E2F6, and GATA1 for a region of chromosome 1 containing the UCHL5 gene (the direction of transcription is shown by the arrow beginning at the start site). E2F4 and E2F6 bind to the promoter region whereas GATA1 binds downstream of the gene. (B) Shown is the analysis of binding sites identified using ChIP-seq for GATA1 and ZNF263 for a region of chromosome 1. The binding sites for these two factors do not cluster at the same genomic locations.
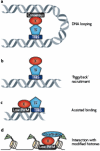
(A) Transcription factor X could bind to its consensus motif (black box) and loop via protein-protein interactions to another transcription factor (TF) bound to a different binding site (TFBS) that is located at a distant region of the chromosome. In this case, because formaldehyde can create both protein-DNA and protein-protein crosslinks, ChIP assays for Factor X would enrich for a region containing its own consensus motif and a region bound by the other factor. (B) Factor X could be recruited to a sequence via protein-protein interactions with another transcription factor (TF) in a manner completely independent of its DNA binding abilities. In this case, ChIP assays would detect binding of Factor X at a region that has no match to its consensus or position weight matrix (PWM). Factor X could bind to a sequence that has a low match to its PWM and be anchored on the genome via protein-protein interactions with a nearby factor (C) or with a specifically modified histone (D). In both of these cases, ChIP assays would detect binding of Factor X at a region that contains a low match to its PWM.
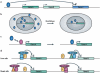
There are a number of reasons, other than a lack of function, why reduction in the level of a transcription factor might not result in a change in expression of the predicted target gene. (A) The transcription factor regulates a gene that is distal to the binding site; therefore, the nearest gene will not show a change in expression upon knockdown of the factor. (B) Knockdown of a factor with an siRNA does not lower the level below that needed for full binding site occupancy; therefore, expression of target genes is not affected. (C) Knockdown of a factor (pink triangle) results in full occupancy by another family member (green triangle) at a site that, under normal conditions, is bound interchangeably by both family members; target gene expression is not affected because the family members are redundant in function. (D) Regulation is dependent on the ubiquitous site-specific factor (yellow oval) in combination with cell type-specific factors. In this example, factor X (yellow oval) is bound to the promoter regions of gene A and gene B in both liver and breast cells and gene A and B are expressed in both tissues. However, in liver, factor X is not involved in regulation of gene B because there is no binding site for the liver-specific factor (blue oval) near the factor X binding site in the gene B promoter. Conversely, in breast cells, factor X regulates gene B through interaction with the breast-specific factor (pink oval) but does not regulate gene A because there is no binding site for the breast-specific factor near the factor X site in the gene A promoter. Thus different subsets of target genes may show changes in expression in different cell types when levels of the ubiquitous site-specific factor are reduced.
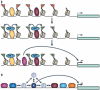
(A) The schematic shows a possible scenario in which two different factors (large yellow and dark red triangles) can bind near to each other on inactive chromatin (represented by the red flags) and each recruit a histone acetyltransferase (HAT), which acetylates histones and creates an open chromatin region (green flags), allowing the binding of another factor (diamond) that stimulates transcription of a gene. In this case, loss of a single factor that recruits a HAT would not result in a major change in regulation of the gene. (B) The schematic shows a possible scenario in which multiple factors (hexagons) bound on either side of factor X (circle) can create a limited search domain for factor X (which is required for activation of a downstream gene). Factor X binds transiently to its binding site; dissociation from the site is followed by localized rebinding and scanning for the high affinity binding site. Transcriptional activation can be enhanced if the scanning is spatially limited by adjacent clusters of other bound factors; loss of a single factor in the cluster would not result in a major change in regulation of the gene.
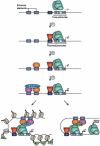
ChIP-chip and ChIP-seq studies have confirmed that RNA polymerase II (RNAPII) and other factors are bound to thousands of promoters that are on at low levels, thus supporting step 1 (as shown in Figure 1). That is, promoter activity can be increased by site-specific DNA binding factors interacting with cis elements in the proximal promoter region and stabilizing the recruitment of the transcriptional machinery through direct interaction of the site-specific factor and the general factors. However, these studies have also revealed that binding of a factor to an enhancer region may be necessary, but not sufficient, for high levels of promoter activity (step 2), thus leading to the inclusion of a new step in the model (step 3): the binding of a cell type-specific partner protein that allows the recruitment of a coactivator to provide for cell type-specific function of a constitutively bound factor. Currently, the projected later steps remain as shown in Figure 1: the enhancer factors can stimulate transcription by (A) recruiting a histone-modifying enzyme to create a more favorable chromatin environment for transcription or (B) recruiting a kinase that can phosphorylate the C terminal domain of RNA polymerase II and stimulate elongation.
Similar articles
-
Genomic location analysis by ChIP-Seq.
Barski A, Zhao K. Barski A, et al. J Cell Biochem. 2009 May 1;107(1):11-8. doi: 10.1002/jcb.22077. J Cell Biochem. 2009. PMID: 19173299 Free PMC article. Review.
-
Mundade R, Ozer HG, Wei H, Prabhu L, Lu T. Mundade R, et al. Cell Cycle. 2014;13(18):2847-52. doi: 10.4161/15384101.2014.949201. Cell Cycle. 2014. PMID: 25486472 Free PMC article. Review.
-
Wei G, Hu G, Cui K, Zhao K. Wei G, et al. Methods Enzymol. 2012;513:297-313. doi: 10.1016/B978-0-12-391938-0.00013-6. Methods Enzymol. 2012. PMID: 22929775
-
Computational discovery of transcriptional regulatory rules.
Pham TH, Clemente JC, Satou K, Ho TB. Pham TH, et al. Bioinformatics. 2005 Sep 1;21 Suppl 2:ii101-7. doi: 10.1093/bioinformatics/bti1117. Bioinformatics. 2005. PMID: 16204087
-
Genomic characterization of perturbation sensitivity.
Ohn JH, Kim J, Kim JH. Ohn JH, et al. Bioinformatics. 2007 Jul 1;23(13):i354-8. doi: 10.1093/bioinformatics/btm172. Bioinformatics. 2007. PMID: 17646317
Cited by
-
Connelly CF, Skelly DA, Dunham MJ, Akey JM. Connelly CF, et al. Mol Biol Evol. 2013 Jul;30(7):1605-13. doi: 10.1093/molbev/mst073. Epub 2013 Apr 25. Mol Biol Evol. 2013. PMID: 23619145 Free PMC article.
-
Microwave assisted synthesis of Py-Im polyamides.
Puckett JW, Green JT, Dervan PB. Puckett JW, et al. Org Lett. 2012 Jun 1;14(11):2774-7. doi: 10.1021/ol3010003. Epub 2012 May 11. Org Lett. 2012. PMID: 22578091 Free PMC article.
-
Transcription factors and splice factors - interconnected regulators of stem cell differentiation.
Mehlferber MM, Kuyumcu-Martinez M, Miller CL, Sheynkman GM. Mehlferber MM, et al. Curr Stem Cell Rep. 2023 Jun;9(2):31-41. doi: 10.1007/s40778-023-00227-2. Epub 2023 Jun 29. Curr Stem Cell Rep. 2023. PMID: 38939410 Free PMC article.
-
Improved predictions of transcription factor binding sites using physicochemical features of DNA.
Maienschein-Cline M, Dinner AR, Hlavacek WS, Mu F. Maienschein-Cline M, et al. Nucleic Acids Res. 2012 Dec;40(22):e175. doi: 10.1093/nar/gks771. Epub 2012 Aug 25. Nucleic Acids Res. 2012. PMID: 22923524 Free PMC article.
-
nMOWChIP-seq: low-input genome-wide mapping of non-histone targets.
Liu Z, Naler LB, Zhu Y, Deng C, Zhang Q, Zhu B, Zhou Z, Sarma M, Murray A, Xie H, Lu C. Liu Z, et al. NAR Genom Bioinform. 2022 Apr 7;4(2):lqac030. doi: 10.1093/nargab/lqac030. eCollection 2022 Jun. NAR Genom Bioinform. 2022. PMID: 35402909 Free PMC article.
References
-
-
Lee TI, Young RA. Transcription of eukaryotic protein-coding genes. Annu. Rev. Genet. 2000;34:77–137. A detailed review of transcriptional regulation, general factors, and accessory proteins that control transcription initation and elongation.
-
-
- Sandelin A, et al. Mammalian RNA polymerase II core promoters: insights from genome-wide studies. Nat Reviews Genetics. 2007;8:424–436. - PubMed
-
-
Consortium TEP. Identification and analysis of functional elements in 1% of the human genome by the ENCODE pilot project. Nature. 2007;447:799–816. Demonstrates how genome-wide studies of transcription, factor binding, chromatin structure, DNA replication, and sequence conservation can synergize.
-
Publication types
MeSH terms
Substances
Grants and funding
LinkOut - more resources
Full Text Sources
Other Literature Sources