ChIP-seq: advantages and challenges of a maturing technology - PubMed
- ️Invalid Date
Review
. 2009 Oct;10(10):669-80.
doi: 10.1038/nrg2641. Epub 2009 Sep 8.
Affiliations
- PMID: 19736561
- PMCID: PMC3191340
- DOI: 10.1038/nrg2641
Review
ChIP-seq: advantages and challenges of a maturing technology
Peter J Park. Nat Rev Genet. 2009 Oct.
Abstract
Chromatin immunoprecipitation followed by sequencing (ChIP-seq) is a technique for genome-wide profiling of DNA-binding proteins, histone modifications or nucleosomes. Owing to the tremendous progress in next-generation sequencing technology, ChIP-seq offers higher resolution, less noise and greater coverage than its array-based predecessor ChIP-chip. With the decreasing cost of sequencing, ChIP-seq has become an indispensable tool for studying gene regulation and epigenetic mechanisms. In this Review, I describe the benefits and challenges in harnessing this technique with an emphasis on issues related to experimental design and data analysis. ChIP-seq experiments generate large quantities of data, and effective computational analysis will be crucial for uncovering biological mechanisms.
Figures
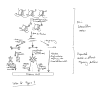
Specific DNA sites that interact with transcription factors or other chromatin-associated proteins as well as sites that correspond to modified chromatin can be profiled using chromatin immunoprecipitation (ChIP) followed by massively parallel sequencing. The ChIP process enriches crosslinked proteins or modified chromatin of interest using an antibody specific to the protein or the histone modification. Purified DNA can be sequenced on any of the next-generation platforms [12]. The basic concepts are similar on these platforms: common adaptors are ligated to the ChIP DNA, and clonally clustered amplicons are generated. The sequencing step involves enzyme-driven extension of all templates in parallel, alternating with detection of florescent labels incorporated with each extension by high-resolution imaging. On the Illumina/Solexa Genome Analyzer (bottom left), clusters of clonal sequences are generated by bridge PCR, and sequencing is performed by sequencing-by-synthesis. On the 454 and SOLiD platforms (bottom middle), clonal sequencing features are generated by emulsion PCR, with amplicons captured to the surface of μm-scale beads. Beads with amplicons are then recovered and immobilized to a planar substrate to be sequenced by pyrosequencing (454) or by DNA ligase-driven synthesis (SOLiD). On single-molecular sequencing platforms such as the HeliScope by Helicos (bottom right), fluorescent nucleotides incorporated into templates can be imaged at the level of single molecules, thus making clonal amplification unnecessary.
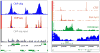
A) An example of ChIP-Seq and ChIP-chip profiles. The figure shows a section of the binding profiles of the chromodomain protein Chromator measured by ChIP-chip (unlogged intensity ratio, blue) and ChIP-Seq (tag density, red) in the D. melanogaster S2 cell line. The tag density profile obtained by ChIP-Seq reveals specific positions of Chromator binding with higher spatial resolution and sensitivity. The ChIP-Seq input DNA (control experiment) tag density is shown (gray) for comparison. B) Examples of different types of ChIP-Seq tag density profiles. Profiles for different types of proteins and histone marks can have different types of features. For example: sharp binding sites, as shown for the insulator binding protein CTCF (red); a mixture of shapes, as shown for RNA Polymerase II (orange), which has a sharp peak followed by a broad region of enrichment; medium size broad peaks, as illustrated by H3K36me3 (green), which is associated with transcription elongation over the gene body; and large domains, as illustrated by H3K27me3 (blue), a repressive markindicative of Polycomb-mediated silencing. Data for part B are from human T-cells, from REF .
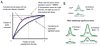
(A) To determine whether enough tags have been sequenced, simulation can be carried out to characterize the fraction of the peaks that would be recovered if a smaller number of tags had been sequenced. In many cases, new statistically significant peaks are discovered at a steady rate with an increasing number of tags (solid curve), i.e., there is no saturation of binding sites. However, when a minimum threshold is imposed for the enrichment ratio between ChIP and input DNA peaks, the rate at which new peaks are discovered slows down (dashed curve). That is, saturation of detected binding sites can occur when sufficiently prominent binding positions are considered. For a given data set, multiple curves corresponding to different thresholds can be examined to identify the threshold at which the curve becomes sufficiently flat to meet the desired saturation criteria (upper right box defined by the red lines). We refer to such threshold as the Minimum Saturation Enrichment Ratio (MSER). MSER can serve as a measure for the depth of sequencing achieved in a data set: A high MSER, for example, indicates that the data set may be under-sampled, as only the more prominent peaks were saturated. See REF Kharchenko et al for details. (B) There are two ways in which a peak can be more statistically significant than another (lower panels compared to upper panels): higher enrichment ratio in ChIP compared to control for the same number of tags (shown under the curve in each case) (lower left) or the same enrichment ratio but a larger number of tag counts (lower right). As the latter case illustrates, there may not be saturation of binding sites when more sequencing leads to less prominent peaks becoming more statistically significant.
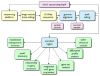
The raw data for ChIP-Seq analysis are images from the next generation sequencing platform (top left). A base-caller converts the image data to sequence tags, which are then aligned to the genome, on some platforms with the aid of quality scores that indicate the reliability of each base call. Peak calling using the ChIP and a control profile (usually input DNA) are used to generate a list of enriched regions ordered by false discovery rate as a statistical measure. Subsequently, the profiles of enriched regions are viewed with a browser and a variety of advanced analyses are performed.
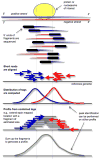
DNA fragments from a chromatin immunoprecipitation experiment are sequenced from the 5′ end. Thus, alignment of these tags to the genome results in two peaks, one on each strand, flanking the location where the protein or nucleosome of interest was bound. This strand-specific pattern can be used for optimal detection of enriched regions. To approximate the distribution of all fragments, each tag location can be extended by an estimated fragment size in the appropriate orientation and the number of fragments is counted
Similar articles
-
Epigenetics meets next-generation sequencing.
Park PJ. Park PJ. Epigenetics. 2008 Nov;3(6):318-21. doi: 10.4161/epi.3.6.7249. Epub 2008 Nov 22. Epigenetics. 2008. PMID: 19098449
-
Mundade R, Ozer HG, Wei H, Prabhu L, Lu T. Mundade R, et al. Cell Cycle. 2014;13(18):2847-52. doi: 10.4161/15384101.2014.949201. Cell Cycle. 2014. PMID: 25486472 Free PMC article. Review.
-
The analysis of ChIP-Seq data.
Ma W, Wong WH. Ma W, et al. Methods Enzymol. 2011;497:51-73. doi: 10.1016/B978-0-12-385075-1.00003-2. Methods Enzymol. 2011. PMID: 21601082
-
ChIPseqR: analysis of ChIP-seq experiments.
Humburg P, Helliwell CA, Bulger D, Stone G. Humburg P, et al. BMC Bioinformatics. 2011 Jan 31;12:39. doi: 10.1186/1471-2105-12-39. BMC Bioinformatics. 2011. PMID: 21281468 Free PMC article.
-
Yan H, Tian S, Slager SL, Sun Z. Yan H, et al. Epigenomics. 2016 Sep;8(9):1239-58. doi: 10.2217/epi-2016-0053. Epub 2016 Jun 20. Epigenomics. 2016. PMID: 27319740 Review.
Cited by
-
Target specificity of the CRISPR-Cas9 system.
Wu X, Kriz AJ, Sharp PA. Wu X, et al. Quant Biol. 2014 Jun;2(2):59-70. doi: 10.1007/s40484-014-0030-x. Quant Biol. 2014. PMID: 25722925 Free PMC article.
-
Jung J, Kim SK, Jung SH, Jeong MJ, Ryu CM. Jung J, et al. Front Microbiol. 2020 Aug 21;11:1978. doi: 10.3389/fmicb.2020.01978. eCollection 2020. Front Microbiol. 2020. PMID: 32973716 Free PMC article.
-
A global genome segmentation method for exploration of epigenetic patterns.
Steiner L, Hopp L, Wirth H, Galle J, Binder H, Prohaska SJ, Rohlf T. Steiner L, et al. PLoS One. 2012;7(10):e46811. doi: 10.1371/journal.pone.0046811. Epub 2012 Oct 12. PLoS One. 2012. PMID: 23077526 Free PMC article.
-
Briggs JA, Mason EA, Ovchinnikov DA, Wells CA, Wolvetang EJ. Briggs JA, et al. Stem Cells Transl Med. 2013 Mar;2(3):175-84. doi: 10.5966/sctm.2012-0117. Epub 2013 Feb 14. Stem Cells Transl Med. 2013. PMID: 23413375 Free PMC article. Review.
-
Herrmann C, Van de Sande B, Potier D, Aerts S. Herrmann C, et al. Nucleic Acids Res. 2012 Aug;40(15):e114. doi: 10.1093/nar/gks543. Epub 2012 Jun 20. Nucleic Acids Res. 2012. PMID: 22718975 Free PMC article.
References
-
- Henikoff S. Nucleosome destabilization in the epigenetic regulation of gene expression. Nat Rev Genet. 2008;9(1):15–26. - PubMed
-
- Li B, et al. The role of chromatin during transcription. Cell. 2007;128(4):707–19. - PubMed
-
- Allis CD, et al., editors. Epigenetics. Cold Spring Harbor Laboratory Press; Cold Spring Harbor, New York: 2007.
Publication types
MeSH terms
Substances
Grants and funding
LinkOut - more resources
Full Text Sources
Other Literature Sources