An improved SUMmOn-based methodology for the identification of ubiquitin and ubiquitin-like protein conjugation sites identifies novel ubiquitin-like protein chain linkages - PubMed
An improved SUMmOn-based methodology for the identification of ubiquitin and ubiquitin-like protein conjugation sites identifies novel ubiquitin-like protein chain linkages
Stanley M Jeram et al. Proteomics. 2010 Jan.
Abstract
Ubiquitin (Ub) and the ubiquitin-like proteins (Ubls) comprise a remarkable assortment of polypeptides that are covalently conjugated to target proteins (or other biomolecules) to modulate their intracellular localization, half-life, and/or activity. Identification of Ub/Ubl conjugation sites on a protein of interest can thus be extremely important for understanding how it is regulated. While MS has become a powerful tool for the study of many classes of PTMs, the identification of Ub/Ubl conjugation sites presents a number of unique challenges. Here, we present an improved Ub/Ubl conjugation site identification strategy, utilizing SUMmOn analysis and an additional protease (lysyl endopeptidase C), as a complement to standard approaches. As compared with standard trypsin proteolysis-database search protocols alone, the addition of SUMmOn analysis can (i) identify Ubl conjugation sites that are not detected by standard database searching methods, (ii) better preserve Ub/Ubl conjugate identity, and (iii) increase the number of identifications of Ub/Ubl modifications in lysine-rich protein regions. Using this methodology, we characterize for the first time a number of novel Ubl linkages and conjugation sites, including alternative yeast (K54) and mammalian small ubiquitin-related modifier (SUMO) chain (SUMO-2 K42, SUMO-3 K41) assemblies, as well as previously unreported NEDD8 chain (K27, K33, and K54) topologies.
Figures
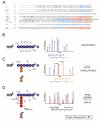
(A) Amino acid sequence alignment of Ub and Ubls. Gene name and species (Homo sapiens (Hs); and Saccharomyces cerevisiae (Sc)) are indicated at left. C-terminal amino acids present in the pro-protein, but absent in the mature protein, are indicated in green. FUB1 and Ub are synthesized as fusion proteins (as indicated by ..…). The Ubl C-terminal “tail” that remains conjugated to a target molecule following trypsin (red text) or LysC (underlined text) digestion is highlighted. Additional residues resulting from LysC digestion are indicated in blue. Lysine residues implicated in Ub/Ubl chain formation (previously published, or as reported here) are indicated in bold. (B) A theoretical unmodified peptide and its corresponding (simplified) CID spectrum. Trypsin cleavage sites indicated by arrows. b- and y-ion series are indicated by dark and light blue peaks, respectively. (C) A theoretical ubiquitylated tryptic peptide, and its corresponding (simplified) CID spectrum. The entire b-ion series, as well as the y5 ion, undergo a +114.04 Da mass shift (shifted ions highlighted in orange). (D) A theoretical Ubl-modified peptide, and its corresponding (simplified) CID spectrum. b’- and y’-ion series denote the fragmentation ion series of the Ubl (in red and pink, respectively). The additional Ubl fragment ions create a highly complex CID spectrum which is uninterpretable by standard database search software. For example, the y’3 ion includes the entire target peptide (and is therefore considered a dependent ion), and would remain unassigned. The b’n-2 ion, derived only from the Ubl tail, is independent of the target peptide. The y2, y3, and y4 ions are unaffected, but would not be assigned by database search software.
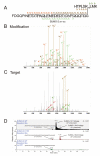
K42-linked SUMO-2 is identified by SUMmOn. (A) Amino acid sequence of the trypsin-digested K42-linked SUMO-2-SUMO-2 peptide, indicating the ion fragments assigned by SUMmOn. (B) SUMmOn-annotated CID spectrum of the K42-linked SUMO-2-SUMO-2 peptide highlighting b’- and y’-ions derived from the modification (i.e. the C-terminal tryptic peptide of SUMO-2, aa 61-92). (C) The same spectrum, highlighting the b- and y-ions derived from the target peptide (aa 37-45 of SUMO-2). (D) Extracted ion chromatograms (EIC) of three different representative SUMO-2 in vitro reaction mixes. The m/z corresponding to the SUMO-2 K11 and K42 linkages elute at ~66 and ~72 min, respectively (top panel). The m/z corresponding to the SUMO-2 K11 linkage is lost when a K11R SUMO-2 mutant protein is used in the in vitro conjugation reaction, while the K42-linked peptide is unaffected (middle panel). The m/z corresponding to the K42 linkage is also lost in a reaction mix lacking ATP (bottom panel). Indicated are (i) the EIC of the SAE2 (SUMO E1) 3+ peptide (R)KPPVPLDWAEVQSQGEETNASDQQNEPQLGLK(D), eluting at ~ 59 min, using an m/z window of 1178.57-1178.59, (ii) an EIC of the 4+ (the most abundant charge state) SUMO-2 K11 linked peptide, using an m/z window of 1291.59-1291.61, and (iii) the EIC of the 4+ SUMO-2 K42 linked peptide (the most abundant charge state), using an m/z window of 1152.29-1152.31. For very large peptides, such as those characterized here, the monoisotopic variant represents only a very small percentage of the peptide population. The m/z windows in Figures 2, 4 and 7 therefore do not bracket the monoisotopic m/z for each linkage, but instead encompass the most abundant isotopic variants (i.e. those that were most often isolated for fragmentation).
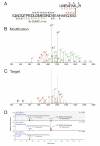
A yeast SUMO K54-linkage is identified using SUMmOn. (A) Amino acid sequence of the K54-linked SUMO peptide, and the fragments assigned by SUMmOn. (B) SUMmOn-annotated CID spectrum of a yeast SUMO conjugate highlighting b’- and y’-ions derived from the C-terminal SUMO tryptic peptide, aa 72-98. (C) the same spectrum, highlighting the b- and y-ions derived from the target peptide (yeast SUMO aa 48-55). (D) EIC of representative in vitro yeast SUMO conjugation reactions with and without ATP. The m/z windows correspond to the 3+ (m/z = 1305.94-1305.96, elution at ~61 min) and 4+ (m/z=974.71-974.73) precursor ions of the SUMO K54-linked peptide. An unmodified yeast SUMO 3+ peptide MSDSEVNQEAKPEVKPEVKPETHINLK(V) (m/z window 1026.52-1026.54, eluting at ~25 min) was detected in both reactions.
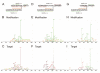
NEDD8 K22, K48 and K54 linkages identified by SUMmOn. (A) Amino acid sequence of the LysC-digested K22-linked NEDD8-NEDD8 peptide, indicating fragments identified using SUMmOn. (B) SUMmOn-annotated CID spectrum of the LysC-digested K22-linked NEDD8-NEDD8 peptide highlighting the b’- and y’-ions derived from the C-terminal LysC fragment of NEDD8, aa 61-76. (C) Identical CID spectrum, highlighting the b- and y-ions derived from the target peptide (NEDD8 aa 12-27). (D-F) As described for A-C, but for the K48-linked NEDD8 peptide. (G-I) As described for A-C, but for the K54-linked NEDD8 peptide. Asterisk (*) denotes pyroglutamate.
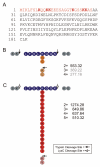
(A) Amino acid sequence of UbcH12/UBE2M. The lysine-rich 28 aa N-terminal extension is highlighted in red, and NEDD8-modified lysine residues identified in this study are in bold. (B and C) Schematic diagram of a NEDD8-modified UbcH12/UBE2M peptide (aa 4-11; K8 modified by NEDD8), cleaved with (B) trypsin (filled arrowhead) or (C) LysC (white arrowhead). Predicted monoisotopic m/z for each peptide at several different charge states are indicated. Grey m/z values fall below those used in typical ion trap mass spectrometer parent ion scans.
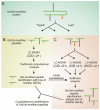
Improved workflow for the identification of Ub/Ubl-conjugation sites. (A) The protein sample of interest is split in two, and digested with trypsin or LysC prior to LC-MS/MS analysis. (B) Standard database searching is used to analyze a portion of the trypsin-digested sample, and to create a subdatabase for SUMmOn. (C) The remainder of the trypsin-digested sample, as well as the LysC-digested sample, are analyzed using SUMmOn. During the initial LC-MS/MS run, CIDs are collected on precursor ions with a charge of 2+ or greater. An additional LC-MS/MS run can be performed in which CIDs are collected on precursor ions with a charge of 4+ or greater to enrich for Ub/Ubl-modified peptides. HCD may also be used to obtain improved fragmentation of highly charged precursor ions. Combining the results of these multiple analyses can generate a comprehensive list of unambiguous Ub/Ubl modification sites.
Similar articles
-
Using mass spectrometry to identify ubiquitin and ubiquitin-like protein conjugation sites.
Jeram SM, Srikumar T, Pedrioli PG, Raught B. Jeram SM, et al. Proteomics. 2009 Feb;9(4):922-34. doi: 10.1002/pmic.200800666. Proteomics. 2009. PMID: 19180541 Review.
-
Xu C, Zhang J, Zhang W, Liu H, Fang J, Xie H. Xu C, et al. Proteomics. 2013 Sep;13(17):2579-84. doi: 10.1002/pmic.201300151. Epub 2013 Aug 9. Proteomics. 2013. PMID: 23828837
-
Structure and evolution of ubiquitin and ubiquitin-related domains.
Burroughs AM, Iyer LM, Aravind L. Burroughs AM, et al. Methods Mol Biol. 2012;832:15-63. doi: 10.1007/978-1-61779-474-2_2. Methods Mol Biol. 2012. PMID: 22350875
-
Ryu KS, Lee KJ, Bae SH, Kim BK, Kim KA, Choi BS. Ryu KS, et al. J Biol Chem. 2003 Sep 19;278(38):36621-7. doi: 10.1074/jbc.M304628200. Epub 2003 Jun 28. J Biol Chem. 2003. PMID: 12832454
-
Probing ubiquitin and SUMO conjugation and deconjugation.
Ovaa H, Vertegaal ACO. Ovaa H, et al. Biochem Soc Trans. 2018 Apr 17;46(2):423-436. doi: 10.1042/BST20170086. Epub 2018 Mar 27. Biochem Soc Trans. 2018. PMID: 29588386 Review.
Cited by
-
Identification of c-MYC SUMOylation by mass spectrometry.
Kalkat M, Chan PK, Wasylishen AR, Srikumar T, Kim SS, Ponzielli R, Bazett-Jones DP, Raught B, Penn LZ. Kalkat M, et al. PLoS One. 2014 Dec 18;9(12):e115337. doi: 10.1371/journal.pone.0115337. eCollection 2014. PLoS One. 2014. PMID: 25522242 Free PMC article.
-
Proteomics of protein post-translational modifications implicated in neurodegeneration.
Ren RJ, Dammer EB, Wang G, Seyfried NT, Levey AI. Ren RJ, et al. Transl Neurodegener. 2014 Oct 30;3(1):23. doi: 10.1186/2047-9158-3-23. eCollection 2014. Transl Neurodegener. 2014. PMID: 25671099 Free PMC article. Review.
-
Nonenzymatic rubylation and ubiquitination of proteins for structural and functional studies.
Singh RK, Sundar A, Fushman D. Singh RK, et al. Angew Chem Int Ed Engl. 2014 Jun 10;53(24):6120-5. doi: 10.1002/anie.201402642. Epub 2014 Apr 24. Angew Chem Int Ed Engl. 2014. PMID: 24764216 Free PMC article.
-
Protein neddylation: beyond cullin-RING ligases.
Enchev RI, Schulman BA, Peter M. Enchev RI, et al. Nat Rev Mol Cell Biol. 2015 Jan;16(1):30-44. doi: 10.1038/nrm3919. Nat Rev Mol Cell Biol. 2015. PMID: 25531226 Free PMC article. Review.
-
Global SUMO Proteome Responses Guide Gene Regulation, mRNA Biogenesis, and Plant Stress Responses.
Mazur MJ, van den Burg HA. Mazur MJ, et al. Front Plant Sci. 2012 Sep 17;3:215. doi: 10.3389/fpls.2012.00215. eCollection 2012. Front Plant Sci. 2012. PMID: 23060889 Free PMC article.
References
-
- Schwartz DC, Hochstrasser M. A superfamily of protein tags: ubiquitin, SUMO and related modifiers. Trends Biochem Sci. 2003;28:321–328. - PubMed
-
- Kerscher O, Felberbaum R, Hochstrasser M. Modification of proteins by ubiquitin and ubiquitin-like proteins. Annu Rev Cell Dev Biol. 2006;22:159–180. - PubMed
-
- Ciechanover A, Iwai K. The ubiquitin system: from basic mechanisms to the patient bed. IUBMB Life. 2004;56:193–201. - PubMed
-
- Hershko A, Ciechanover A. The ubiquitin system. Annu Rev Biochem. 1998;67:425–479. - PubMed
Publication types
MeSH terms
Substances
LinkOut - more resources
Full Text Sources
Molecular Biology Databases
Research Materials
Miscellaneous