Phenotypic transition of the collecting duct epithelium in congenital urinary tract obstruction - PubMed
Phenotypic transition of the collecting duct epithelium in congenital urinary tract obstruction
Peter Trnka et al. J Biomed Biotechnol. 2010.
Abstract
Epithelial-mesenchymal transition (EMT) has emerged in recent years as an important process in the development of organ fibrosis in many human diseases. Our previous experience in a nonhuman primate model of obstructive nephropathy suggested that EMT of collecting duct epithelium contributes to the development of interstitial fibrosis. In this study we demonstrate for the first time in humans that obstructed fetal collecting duct epithelium undergoes transition to mesenchymal phenotype, characterized by decreased expression of epithelial markers, de novo expression of mesenchymal markers with subsequent loss of cell-cell interaction, disruption of the basement membrane, and increased deposition of extracellular matrix into the expanded interstitium of the obstructed kidney. The results of this study therefore support the previous findings from animal studies and suggest that EMT of the collecting duct epithelium might contribute to the development of interstitial fibrosis in human fetal obstructive nephropathy.
Figures
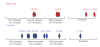
Gestational and postnatal ages of the obstructed and control kidney tissue samples.
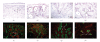
Histological features of human fetal obstructive nephropathy. Obstructed 18 weeks gestation kidney shows disorganized cortical architecture with multiple glomerular cysts (c), decreased glomerular (g) endowment ((b) and (f)), and reduced number and dilation of the collecting ducts (cd) in the hypoplastic medulla ((d) and (h)). Cortex ((a) and (e)) and medulla ((c) and (g)) of a normal 18-week gestation kidney. (a)–(d): hematoxylin and eosin stain; (e)–(h) immunofluorescence histochemistry: green = cytokeratin, red = vimentin. Scale bar = 100 μm.
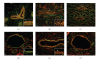
E-cadherin and β -catenin immunoreactivity (IR). Normal kidneys ((a)–(c)). (a) In the early gestation kidney (10 weeks), E-cadherin-IR (red) and β-catenin-IR (green) are localized in the cytoplasm (arrows). (b) Colocalization of E-cadherin-IR and β-catenin-IR (yellow) to the intercellular junctions is well established by late gestation (27 weeks) (arrows), and (c) persists in the normal postnatal (12 months) kidney (arrow). Obstructed kidneys ((d)–(f)). (d) Disruption of E-cadherin-IR and β-catenin-IR colocalization at the intercellular junctions, with translocation of β-catenin-IR to the cytoplasm (green) and of E-cadherin-IR to the nucleus (red) (arrows in inset) in the (d) mid gestation kidney (18 weeks), (e) late gestation kidney (36 weeks), and (f) postnatally (16 months kidney). Scale bar = 25 μm.
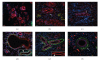
E-cadherin and α-smooth muscle actin immunoreactivity. Normal kidneys ((a)–(c)). In the normal mid gestation (18 weeks) (a), late gestation (27 weeks) (b), and postnatal (12 months) (c) kidneys, α-smooth muscle actin (α-SMA)-IR (green) was absent in the CD epithelium; however, intercellular localization of E-cadherin-IR (red) was well established from mid gestation onwards (arrows). Obstructed kidneys ((d)–(f)). Circumferential collars expressing α-SMA-IR (green) are present in the (d) mid gestation (18 weeks), (e) late gestation (36 weeks), and (f) postnatal (16 months) kidneys (arrows). De novo expression of α-SMA-IR in the obstructed CD epithelium is present at 18 weeks gestation ((d) inset, arrowhead) but not in the late gestation (e) or postnatal (f) kidneys. As in Figure 3, E-cadherin expression (red) is disrupted, with translocation from the cell membrane to cytoplasm ((d)–(f)) and to the nucleus ((e) inset, arrowhead). Scale bar = 25 μm.
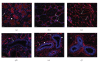
Vimentin and collagen IV immunoreactivity. Normal kidneys ((a)–(c)). (a) Interstitial expression of vimentin-IR (blue) (arrow) and diffuse cytoplasmic expression of collagen IV (ColIV)-IR (red) (arrowhead) are present in the mid gestation (18 weeks) kidney. (b) Thin, regular, well-defined expression of ColIV-IR in the CD basement membranes (arrows) is well established by late gestation (27 weeks), and (c) persists postnatally (12 months) (arrows). Obstructed kidneys ((d)–(f)). (d) De novo basolateral expression of vimentin-IR (blue) (arrow) in the CD epithelium of the obstructed 18 weeks gestation kidney. (e) More pronounced vimentin-IR expression (arrowheads) and disruption of ColIV-IR expression (red) (arrows) in the CD basement membrane of the late gestation (36 weeks) obstructed CD epithelium, and (f) in the obstructed postnatal (16 months) kidneys. Deposition of ColIV-IR in the expanded interstitium (asterisk), and in the periductal collars (plus) is also present. Scale bar = 25 μm.
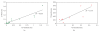
Correlation of the severity of CD dilatation with periductal collar formation and with CD epithelial vimentin expression. The relationship between CD dilatation (x-axis), and the areas of periductal collars expressing α-SMA (a) and of CD epithelium expressing vimentin (b) (y-axis) is shown. Measurements were performed in obstructed fetal (36 weeks gestation) and postnatal (16 months) kidneys (n = 3). All areas are expressed in mm2.
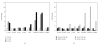
Gene expression in normal and obstructed fetal kidneys. (a) Quantitative PCR analysis of total RNA extracted from 10, 15, and 18 weeks gestation normal kidneys, for genes encoding epithelial-specific (E-cad, N-cad, α-cat, and β-cat) and mesenchymal-specific (vimentin, ColIVa3, ColIVa5 and α-SMA) proteins. Values are expressed as fold change in mRNA expression compared to the normal 10 weeks gestation kidney as reference. (b) Quantitative PCR analysis of total RNA extracted from obstructed kidneys at 18 weeks gestation (mild and severe) and 36 weeks gestation (severe), and from 18 weeks normal kidney. Values are expressed as fold change in mRNA expression compared to the normal 18 weeks gestation kidney as reference.
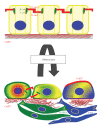
Paradigm of changes associated with human congenital urinary tract obstruction. The obstruction of the developing kidneys causes dissociation of the cytoskeletal proteins (E-cadherin, β-catenin) and their translocation to the cytoplasm and the nucleus. Affected cells become flattened and start expressing mesenchymal markers (vimentin, α-SMA) giving them more flexibility and contractility. Breakdown of the ductal basement membrane allows the cells to migrate into the interstitium and to contribute to the myoepthelial population of periductal cells.
Similar articles
-
Collecting duct epithelial-mesenchymal transition in fetal urinary tract obstruction.
Butt MJ, Tarantal AF, Jimenez DF, Matsell DG. Butt MJ, et al. Kidney Int. 2007 Oct;72(8):936-44. doi: 10.1038/sj.ki.5002457. Epub 2007 Aug 1. Kidney Int. 2007. PMID: 17667982
-
Remodeling of the fetal collecting duct epithelium.
Hiatt MJ, Ivanova L, Toran N, Tarantal AF, Matsell DG. Hiatt MJ, et al. Am J Pathol. 2010 Feb;176(2):630-7. doi: 10.2353/ajpath.2010.090389. Epub 2009 Dec 24. Am J Pathol. 2010. PMID: 20035053 Free PMC article.
-
Direct contribution of epithelium to organ fibrosis: epithelial-mesenchymal transition.
Guarino M, Tosoni A, Nebuloni M. Guarino M, et al. Hum Pathol. 2009 Oct;40(10):1365-76. doi: 10.1016/j.humpath.2009.02.020. Epub 2009 Aug 19. Hum Pathol. 2009. PMID: 19695676 Review.
-
Perinatal obstructive nephropathy.
Chevalier RL. Chevalier RL. Semin Perinatol. 2004 Apr;28(2):124-31. doi: 10.1053/j.semperi.2003.11.009. Semin Perinatol. 2004. PMID: 15200251 Review.
-
Hajarnis S, Yheskel M, Williams D, Brefort T, Glaudemans B, Debaix H, Baum M, Devuyst O, Patel V. Hajarnis S, et al. J Am Soc Nephrol. 2018 Feb;29(2):518-531. doi: 10.1681/ASN.2017030334. Epub 2017 Oct 11. J Am Soc Nephrol. 2018. PMID: 29021386 Free PMC article.
Cited by
-
Urinary biomarkers in obstructive nephropathy.
Trnka P, Ivanova L, Hiatt MJ, Matsell DG. Trnka P, et al. Clin J Am Soc Nephrol. 2012 Oct;7(10):1567-75. doi: 10.2215/CJN.09640911. Epub 2012 Aug 2. Clin J Am Soc Nephrol. 2012. PMID: 22859744 Free PMC article.
-
Li J, Ariunbold U, Suhaimi N, Sunn N, Guo J, McMahon JA, McMahon AP, Little M. Li J, et al. J Am Soc Nephrol. 2015 Jan;26(1):81-94. doi: 10.1681/ASN.2013050517. Epub 2014 Jun 5. J Am Soc Nephrol. 2015. PMID: 24904087 Free PMC article.
-
Convento M, Pessoa E, Aragão A, Schor N, Borges F. Convento M, et al. Oncotarget. 2019 Feb 1;10(10):1102-1118. doi: 10.18632/oncotarget.26634. eCollection 2019 Feb 1. Oncotarget. 2019. PMID: 30800221 Free PMC article.
-
Wt-1 Expression Linked to Nitric Oxide Availability during Neonatal Obstructive Nephropathy.
Mazzei L, Manucha W. Mazzei L, et al. Adv Urol. 2013;2013:401750. doi: 10.1155/2013/401750. Epub 2013 Oct 31. Adv Urol. 2013. PMID: 24288526 Free PMC article. Review.
-
Mediators and mechanisms of heat shock protein 70 based cytoprotection in obstructive nephropathy.
Mazzei L, Docherty NG, Manucha W. Mazzei L, et al. Cell Stress Chaperones. 2015 Nov;20(6):893-906. doi: 10.1007/s12192-015-0622-z. Epub 2015 Jul 31. Cell Stress Chaperones. 2015. PMID: 26228633 Free PMC article. Review.
References
-
- Smith JM, Stablein DM, Munoz R, Hebert D, McDonald RA. Contributions of the transplant registry: the 2006 annual report of the north american pediatric renal trials and collaborative studies (NAPRTCS) Pediatric Transplantation. 2007;11(4):366–373. - PubMed
-
- Hay ED, Zuk A. Transformations between epithelium and mesenchyme: normal, pathological, and experimentally induced. American Journal of Kidney Diseases. 1995;26(4):678–690. - PubMed
-
- Liu Y. Epithelial to mesenchymal transition in renal fibrogenesis: pathologic significance, molecular mechanism, and therapeutic intervention. Journal of the American Society of Nephrology. 2004;15(1):1–12. - PubMed
-
- Zeisberg M, Kalluri R. The role of epithelial-to-mesenchymal transition in renal fibrosis. Journal of Molecular Medicine. 2004;82(3):175–181. - PubMed