Chromosome territories - PubMed
Review
Chromosome territories
Thomas Cremer et al. Cold Spring Harb Perspect Biol. 2010 Mar.
Abstract
Chromosome territories (CTs) constitute a major feature of nuclear architecture. In a brief statement, the possible contribution of nuclear architecture studies to the field of epigenomics is considered, followed by a historical account of the CT concept and the final compelling experimental evidence of a territorial organization of chromosomes in all eukaryotes studied to date. Present knowledge of nonrandom CT arrangements, of the internal CT architecture, and of structural interactions with other CTs is provided as well as the dynamics of CT arrangements during cell cycle and postmitotic terminal differentiation. The article concludes with a discussion of open questions and new experimental strategies to answer them.
Figures
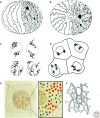
Early concepts in favor of a territorial organization of chromosomes in interphase nuclei. (A–B) Carl Rabĺs hypothetical view (Rabl 1885) of a territorial chromosome arrangement in the interphase nucleus was based on studies of Proteus and Salamandra, in particular on epithelial cells from Salamandra maculata larvae. (A) Side view; supposed CTs are built up from primary chromatin threads (left side), from which secondary and tertiary threads branch out and form a chromatin network (right side). Spindle attachment sites, now known as centromeres, congress at one site of the nucleus (top, Rabl’s Polfeld), whereas the telomeres cluster at the opposite site (bottom, Rabl’s Gegenpolseite). (B) View from above on the Polfeld. (C) Drawings made by Theodor Boveri (1909) from two Ascaris megalocephala univalens embryos fixed during prophase of the two-cell stage. Arrows point to nuclear protrusions containing the distal parts of the two germ line chromosomes. (D) Boveri’s drawing of a fixed four-cell embryo shows two pairs of cells with a distinctly different arrangement of these protrusions in interphase nuclei. Boveri argued that the two upper and two lower cells, respectively, represent daughter cells and explained the strikingly different nuclear protrusion patterns observed in the two cell pairs as a result of chromosome movements during prometaphase. (E) Eduard Strasburger's colored model view of a tissue cell nucleus from the plant Galtonia candicans (Strasburger 1905). Chromosome territories supposedly are built up from higher‐order chromatin foci delineated in red and blue. (F) The white and gray shaded bundles in Boveri’s sketch from 1909 reflect two neighboring CTs with sponge-like structures built up from networks of anastomizing chromatin bundles. The continuous lines reflect Rabl’s primary threads, and the dotted line depicts the possibility of a rare pathological situation, in which secondary chromatin threads from one CT encompass the primary thread of the other, a situation possibly leading to an exchange of chromatin material between the chromosomes or a segregation failure during the next mitosis.
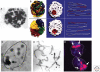
Experimental evidence for a territorial organization of interphase chromosomes. (A) Giemsa stained interphase nucleus of a fixed Chinese hamster cell (CHO line) reveals chromatin clumps likely representing individual CTs (reprinted with permission from Stack et al. 1977). (B) Experimental rationale of laser-UV-microbeam experiments to distinguish between a nonterritorial (upper row) and a territorial (bottom row) chromosome arrangement in cell nuclei (compare Cremer et al. 1982a). (C) Autoradiograph of a diploid Chinese Hamster cell. The nucleus of the living cell was microirradiated in G1, pulse-labeled with 3H thymidine and fixed immediately thereafter. The arrow points to a cluster of silver grains detected over the site of microirradiation. (D) Metaphase spread from the same experiment obtained about 40 hours after microirradiation. One chromosome 1 and one chromosome 2 are intensely marked with silver grains, indicating that the microbeam hit the respective territories during interphase, whereas their homologs are unlabeled, arguing against the spatial association of the homolog territories. (E) Immunocytochemical identification of microirradiated DNA (arrows) in a pair of Chinese hamster daughter nuclei fixed around 4 hours after microirradiation of a small part of a metaphase plate. The mirror-like distribution of microirradiated chromatin in the two daughter nuclei argues for a similar arrangement of chromosome territories (reprinted from Cremer et al. [1984b] with permission).
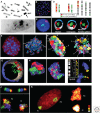
Direct evidence for chromosome territories (CTs) by in situ hybridization experiments. (A) In situ hybridization with biotinylated human genomic DNA of a Chinese hamster × human hybrid cell line carrying a single human X chromosome reveals the specifically labeled human X chromosome (arrow) in metaphase spreads (top; other chromosomes are Giemsa stained) and its respective human X territory in interphase nuclei (bottom); for details, see Schardin et al. (1985). (B) Visualization of individual chromosomes in a human (HSA) metaphase plate (chr. HSA18 in red, chr. HSA 19 in green) after fluorescence in situ hybridization (FISH) using labeled chromosome painting probes. (C) A single light optical mid-section through the nucleus of a human lymphoblastoid cell after 3D FISH with the same painting probes shows a HSA 19 CT (green) in the nuclear center and a HSA 18 CT (red) at the periphery; for details, see Tanabe et al. (2002). (D) Top: Idiogramatic illustration of primate chromosomes or subchromosomal regions from the Orangutan (pongus pygmaeus, PPY, middle) and white handed gibbon (Hylobates lar, HLA, right) orthologous to human chromosomes 18 (red) and 19 (green). Note the pronounced chromosomal rearrangements between the human and the HLA karyotype: The arm of a large HLA chromosome is orthologous to HSA 18, whereas four HSA 19 orthologous chromosome segments are distributed on three other large HLA chromosomes. Bottom: 3D reconstructions of representative HSA (left), PPY (middle), and HLA (right) lymphoblastoid cell nuclei reveal the same nonrandom radial nuclear positions of orthologous gene dense HSA 19 (green) and gene poor chromatin HSA 18 (red); for details, including quantitative evaluation, see Tanabe et al. (2002). (E) Painted CTs of the gene dense chromosome BTA 19 (green) and gene poor BTA 20 chromosome (red) in a domestic cattle (Bos taurus, BTA) embryo during blastocyst stage. Left: Maximum intensity projections of painted CTs in DAPI stained nuclei (blue). Right: 3D reconstructions of nuclei of the same embryo from a different perspective. Gene dense BTA 19 chromatin is preferentially distributed in the nuclear interior and gene poor BTA 20 chromatin at the nuclear periphery: for details, including quantitative evaluation, see Koehler et al. (2009). (F) Simultaneous delineation of all chromosomes in a human fibroblast nucleus (left) and a prometaphase rosette (right) by multi-color FISH. Light optical mid-sections with false color representation of all CTs and prometaphase chromosomes, respectively, are shown. Examples of individual CTs and mitotic chromosomes are denoted with their respective karyotypic number; for details, see Bolzer et al. (2005). (G) Partial 3D reconstruction of a human lymphocyte nucleus after 3D-FISH of two differently labeled sets of BAC clones from HSA 12 carrying sequences from several gene-dense (green) and several gene-poor chromosome segments (red), respectively. This nucleus illustrates two neighboring HSA 12 CTs with distinct gene density correlated radial nuclear arrangement. (H) Left: 3D reconstruction of a single painted HSA 12 territory (blue) showing the distinctly different, polarized arrangements of these two sets of BAC-clones. Right: 3D reconstruction of a HSA 12 CT recorded after 3D FISH with two differentially labeled sets of BAC clones containing sequences from highly expressed (green) and repressed genes (red), respectively, shows that active and silent genes are distributed throughout the CT; for details, see Kupper et al. (2007). (I) Multicolor 3D FISH to a human fibroblast reveals the two HSA 11 CTs (blue) together with the particular gene dense region 11p15.5 (green, yellow, and red). For the delineation of this region, 15 BACs were used and differentially labeled as shown in the inset. Z-projections of light optical serial sections illustrate different shapes of this region: a finger-like chromatin protrusion in the lower CT and a much more compact shape in the upper CT; for details, see Albiez et al. (2006). (J) Top: Two human X chromosomes in a human fibroblast metaphase plate are shown after multicolor FISH with four differentially labeled BAC pools representing four segments from qter to pter (q-arm: green, blue; p-arm: yellow, red). Bottom: Projections of light optical sections through the Xa- and Xi-territory of a human fibroblast nucleus following 3D FISH with the same BAC pools show four separate domains of these segments within the Xa- and Xi-territory (images courtesy of Kathrin Teller, Univ of Munich). (K) Left: Painting of the Xa- and Xi-territory in a female human fibroblast nucleus (46,XX) exemplifies the different shape and painting intensity of the two X-territories. The Xi-territory was independently identified by Barr body staining (not shown). Right: intensity profiles of enlarged images of the Xi- and Xa-territory. The color code white, yellow, and red reflects high, medium, and low intensities (images courtesy of Irina Solovei, Univ of Munich).
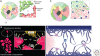
Different models of nuclear architecture. (A) Chromosome territory‐interchromatin compartment (CT-IC) model (for description, see text). (B) Hypothetical view of the functional nuclear architecture according to the CT-IC model. Chromatin domains are considered the major constituents of a CT. The IC expands between these domains as a rather DNA free nuclear compartment carrying splicing speckles and nuclear bodies. The width of the IC space is highly variable depending on Brownian movements of chromatin domains and allowing transient contacts of domain surfaces in cis and trans. During ongoing transcription, genes are at least partially decondensed at any given time into the perichromatin region (PR) located at the domain periphery. Perichromatin fibrils (PF) are generated there. Each PF carries a nascent transcript (green) from a different gene. White dots with a line symbolize RNA Pol II molecules with their CTD domain, which may play a role in the structural organization of splicing events. Splicing speckles located in the IC provide the splicing factors to PFs, which also represent the structures in which cotranscriptional splicing occurs. (C) According to the interchromatin network (ICN) model (Branco and Pombo 2006), intermingling chromatin fibers/loops from the same CT, as well as from neighboring CTs, can make contact in cis and trans. Blue dots represent sites of intrachromosomal and interchromosomal contacts with unknown composition. Although there is extensive space between chromatin fibers/loops, this space should not be confused with the functional relationship of the IC and PR predicted by the CT-IC model. (D) Model suggested by Fraser and Bickmore (Fraser and Bickmore 2007, figure reprinted with permission from Macmillan Publishers Ltd). These authors review evidence arguing for the colocalization of genes in the nucleus for expression or coregulation. Transcription factories (dark pink) can recruit genes in cis and trans located on decondensed chromatin loops that extend outside chromosome territories. The pale pink area on the left represents a splicing-factor enriched speckle. The blue circle exemplifies an interaction for coregulation in trans, which can occur between regulatory elements and/or gene loci.
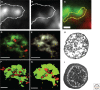
Experimental evidence against and in favor of an interchromatin space. Fluorescence microscopic images of a painted HSA 5 CT (A) and HSA 7 CT (B) in a cryosection of approximately 150 nm thickness from a human lymphocyte nucleus (Branco and Pombo 2006). (C) Overlap of images A and B shows the two CTs in different colors. The area of assumed intermingling between the two CTs is delineated by the yellow line. (D–G) Topographical relationships between double minute (DM) chromosomes carrying active MYCN genes (red) and the painted 3q-arm domain (green) observed in a nucleus of the human neuroblastoma cell HDN-16 (for details, see Solovei et al. 2000). (D) Laser confocal section. DMs denoted by 1–5 are located both at the periphery of the 3q-arm domain and in channel-like invaginations of the interchromatin compartment (IC) penetrating into the interior of the arm domain. (E) IC invaginations are emphasized in this gray image of the same 3q-arm domain section. Numbers indicate the location of DMs 1–5 in D. (F–G) 3D reconstruction of the entire 3q-domain (green) with all associated DMs (red). (F) top-view and (G) section through the reconstructed domain. (H,I) 3D reconstruction of a rat liver cell nucleus specifically stained for DNA and imaged by Serial Block-Face—Scanning Electron Microscopy (Rouquette et al. 2009). (H) A reconstructed mid part of the nucleus with 250-nm thickness shows a strongly inhomogeneous distribution of DNA in higher-order chromatin clusters (gray) and the wide mostly DNA free interchromatin compartment (white) expanding between these clusters. (I) Reconstruction of the major part of the nucleus. The dotted line indicates the removal of the nuclear periphery exposed to the viewer. Here, the nuclear volume seems to be entirely filled with chromatin, most likely—as we suggest—as a consequence of the sponge-like organization of CTs built up from interconnected chromatin domains/bundles permeated by the IC.
Similar articles
-
[Topology of chromosomes in somatic cells. Part 1].
Zegało M, Wiland E, Kurpisz M. Zegało M, et al. Postepy Hig Med Dosw (Online). 2006;60:331-42. Postepy Hig Med Dosw (Online). 2006. PMID: 16819432 Review. Polish.
-
[The progress and research of chromosome territories (CTs)].
Fu ML, Li ZY, Hu FF, Huang SF. Fu ML, et al. Yi Chuan. 2006 Feb;28(2):236-42. Yi Chuan. 2006. PMID: 16520323 Review. Chinese.
-
Nuclear architecture and the induction of chromosomal aberrations.
Cremer C, Münkel C, Granzow M, Jauch A, Dietzel S, Eils R, Guan XY, Meltzer PS, Trent JM, Langowski J, Cremer T. Cremer C, et al. Mutat Res. 1996 Nov;366(2):97-116. doi: 10.1016/s0165-1110(96)90031-7. Mutat Res. 1996. PMID: 9001577 Review.
-
Cremer T, Cremer C. Cremer T, et al. Eur J Histochem. 2006 Jul-Sep;50(3):161-76. Eur J Histochem. 2006. PMID: 16920639 Review.
-
Organization and dynamics of plant interphase chromosomes.
Schubert I, Shaw P. Schubert I, et al. Trends Plant Sci. 2011 May;16(5):273-81. doi: 10.1016/j.tplants.2011.02.002. Epub 2011 Mar 9. Trends Plant Sci. 2011. PMID: 21393049 Review.
Cited by
-
Multiscale molecular modeling of chromatin with MultiMM: From nucleosomes to the whole genome.
Korsak S, Banecki K, Plewczynski D. Korsak S, et al. Comput Struct Biotechnol J. 2024 Oct 2;23:3537-3548. doi: 10.1016/j.csbj.2024.09.025. eCollection 2024 Dec. Comput Struct Biotechnol J. 2024. PMID: 39435339 Free PMC article.
-
Delint-Ramirez I, Konada L, Heady L, Rueda R, Jacome ASV, Marlin E, Marchioni C, Segev A, Kritskiy O, Yamakawa S, Reiter AH, Tsai LH, Madabhushi R. Delint-Ramirez I, et al. Mol Cell. 2022 Oct 20;82(20):3794-3809.e8. doi: 10.1016/j.molcel.2022.09.012. Epub 2022 Oct 6. Mol Cell. 2022. PMID: 36206766 Free PMC article.
-
Chromosome conformation of human fibroblasts grown in 3-dimensional spheroids.
Chen H, Comment N, Chen J, Ronquist S, Hero A, Ried T, Rajapakse I. Chen H, et al. Nucleus. 2015;6(1):55-65. doi: 10.1080/19491034.2014.1003745. Nucleus. 2015. PMID: 25738643 Free PMC article.
-
A critical role for X-chromosome architecture in mammalian X-chromosome dosage compensation.
Dror I, Tan T, Plath K. Dror I, et al. Curr Opin Genet Dev. 2024 Aug;87:102235. doi: 10.1016/j.gde.2024.102235. Epub 2024 Jul 25. Curr Opin Genet Dev. 2024. PMID: 39053028 Review.
-
Higher-order chromatin structure: bridging physics and biology.
Fudenberg G, Mirny LA. Fudenberg G, et al. Curr Opin Genet Dev. 2012 Apr;22(2):115-24. doi: 10.1016/j.gde.2012.01.006. Epub 2012 Feb 22. Curr Opin Genet Dev. 2012. PMID: 22360992 Free PMC article. Review.
References
-
- Akhtar A, Gasser SM 2007. The nuclear envelope and transcriptional control. Nat Rev Genet 8:507–517 - PubMed
-
- Alberts B, Johnson A, Lewis J, Raff M, Roberts K, Walter P 2008. Molecular biology of the cell Garland Science, New York
-
- Albiez H, Cremer M, Tiberi C, Vecchio L, Schermelleh L, Dittrich S, Kupper K, Joffe B, Thormeyer T, von Hase J, et al.2006. Chromatin domains and the interchromatin compartment form structurally defined and functionally interacting nuclear networks. Chromosome Res 14:707–733 - PubMed
Publication types
MeSH terms
LinkOut - more resources
Full Text Sources
Other Literature Sources
Research Materials