Ascending projections from the caudal visceral nucleus of the solitary tract to brain regions involved in food intake and energy expenditure - PubMed
- ️Fri Jan 01 2010
Review
Ascending projections from the caudal visceral nucleus of the solitary tract to brain regions involved in food intake and energy expenditure
Linda Rinaman. Brain Res. 2010.
Abstract
Metabolic homeostasis reflects the complex output of endocrine, autonomic, and behavioral control circuits that extend throughout the central nervous system. Brain regions that control food intake and energy expenditure are privy to continuous visceral sensory feedback signals that presumably modulate appetite, satiety, digestion, and metabolism. Sensory signals from the gastrointestinal tract and associated digestive viscera are delivered to the brain primarily by vagal afferents that terminate centrally within the caudal nucleus of the solitary tract (NST), with signals subsequently relayed to higher brain regions by parallel noradrenergic and peptidergic projection pathways arising within the NST. This article begins with an overview of these ascending pathways identified in adult rats using a standard anterograde tracer microinjected into the caudal visceral sensory region of the NST, and also by immunocytochemical localization of glucagon-like peptide-1. NST projection targets identified by these two approaches are compared to the distribution of neurons that become infected after inoculating the ventral stomach wall with a neurotropic virus that transneuronally infects synaptically-linked chains of neurons in the anterograde (i.e., ascending sensory) direction. Although the focus of this article is the anatomical organization of axonal projections from the caudal visceral NST to the hypothalamus and limbic forebrain, discussion is included regarding the hypothesized role of these projections in modulating behavioral arousal and coordinating endocrine and behavioral (i.e., hypophagic) responses to stress.
2010 Elsevier B.V. All rights reserved.
Figures
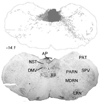
Iontophoretic PhAL injection site within the caudal DVC in an adult male Sprague-Dawley rat. The dark gray shaded area in the upper panel depicts the region of the tracer injection site, which contained PhAL immunoperoxidase labeling that was too dense to accurately draw. See Figure 2 for immunofluorescence labeling of PhAL-concentrating neurons in an adjacent tissue section. Labeled fibers throughout the rest of the section in the upper panel (and in Figures 3–10) arise from PhAL-concentrating neurons located within the injection site. The lower panel is a nearby Nissl-stained tissue section from the same rat. The approximate rostro-caudal level of each section (relative to bregma, in mm) is indicated, based on a standard rat brain atlas (Swanson, 2004). See Table 1 for abbreviations.
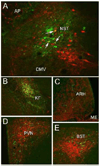
Dual immunofluorescent localization of PhAL (green) and the noradrenergic synthetic enzyme, DbH (red). A: Individual NST neurons concentrating PhAL (green) within the iontophoretic tracer injection site (see Figure 1). A subset of these PhAL-positive neurons are DbH-positive (arrows point out 3 examples). B: PhAL-labeled fibers within the KF subregion of the lateral parabrachial nucleus. C: PhAL-labeled fibers within the hypothalamic ARH. D: PhAL-labeled fibers within the PVN. E: PhAL-labeled fibers within the BST. Note that each photomicrograph depicts PhAL and DbH immunofluorescent labeling photographed at only one focal plane through the section. See Table 1 for abbreviations.
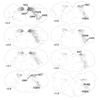
Anterogradely transported PhAL from the caudal DVC (see Figures 1 and 2A for injection site) to more rostral regions of the medulla. The approximate rostro-caudal level of each section (relative to bregma, in mm) is indicated, based on a standard rat brain atlas (Swanson, 2004). Upper left = caudal, lower right = rostral. See Table 1 for abbreviations.
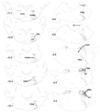
Anterogradely transported PhAL from the caudal DVC (see Figures 1 and 2A for injection site) to the pons. The approximate rostro-caudal locations of each section relative to bregma are indicated, based on a standard rat brain atlas (Swanson, 2004). Upper left = most caudal, lower right = most rostral. See Table 1 for abbreviations.
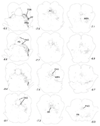
Anterogradely transported PhAL from the caudal DVC (see Figures 1 and 2A for injection site) to more rostral regions of the pons and midbrain. The approximate rostro-caudal level of each section (relative to bregma, in mm) is indicated, based on a standard rat brain atlas (Swanson, 2004). Upper left = caudal, lower right = rostral. See Table 1 for abbreviations.
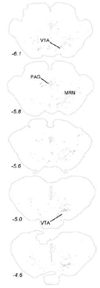
Anterogradely transported PhAL from the caudal DVC (see Figures 1 and 2A for injection site) to more rostral regions of the midbrain and caudal hypothalamus. The approximate rostro-caudal level of each section (relative to bregma, in mm) is indicated, based on a standard rat brain atlas (Swanson, 2004). Top = caudal, bottom = rostral. See Table 1 for abbreviations.
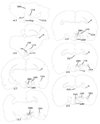
Anterogradely transported PhAL from the caudal DVC (see Figures 1 and 2A for injection site) to the hypothalamus and amygdala. The approximate rostro-caudal level of each section (relative to bregma) is indicated, based on a standard rat brain atlas (Swanson, 2004). Upper left = caudal, lower right = rostral. See Table 1 for abbreviations.
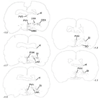
Anterogradely transported PhAL from the caudal DVC (see Figures 1 and 2A for injection site) to more rostral regions of the hypothalamus and amygdala. The approximate rostro-caudal level of each section (relative to bregma, in mm) is indicated, based on a standard rat brain atlas (Swanson, 2004). Upper left = caudal, lower right = rostral. See Table 1 for abbreviations.
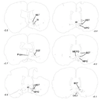
Anterogradely transported PhAL from the caudal DVC (see Figures 1 and 2A for injection site) to more rostral regions of the amygdala, substantia innominata, and bed nucleus of the stria terminalis. The approximate rostro-caudal level of each section (relative to bregma, in mm) is indicated. Upper left = caudal, lower right = rostral. See Table 1 for abbreviations.
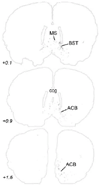
Anterogradely transported PhAL from the caudal DVC (see Figures 1 and 2A for injection site) to more rostral regions of the bed nucleus of the stria terminalis and nucleus accumbens. The approximate rostro-caudal level of each section (relative to bregma, in mm) is indicated, based on a standard rat brain atlas (Swanson, 2004). Top = caudal, bottom = rostral. See Table 1 for abbreviations.
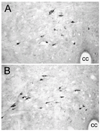
GLP-1 immunoperoxidase labeling of neuron cell bodies within the caudal medullary DVC (A and B, approximately 15.0 and 14.0 mm caudal to bregma, respectively). See Table 1 for abbreviations.
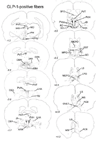
Distribution of GLP-1-immunopositive fibers within the diencephalon and limbic forebrain. The approximate rostro-caudal level of each section (relative to bregma, in mm) is indicated, based on a standard rat brain atlas (Swanson, 2004). Top = caudal, bottom = rostral. See Table 1 for abbreviations.
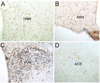
GLP-1-positive fibers (brown) within the hypothalamic DMH (A), ARH (B), and PVH (C), and within the ventral striatum (D). Blue-black nuclear Fos immunolabeling is the result of lithium chloride (0.15M, 1% BW, i.p.) administration 90 min before perfusion fixation. See Table 1 for abbreviations.
Similar articles
-
Kreisler AD, Davis EA, Rinaman L. Kreisler AD, et al. Physiol Behav. 2014 Sep;136:47-54. doi: 10.1016/j.physbeh.2014.01.015. Epub 2014 Feb 6. Physiol Behav. 2014. PMID: 24508750 Free PMC article.
-
Synaptic Inputs to the Mouse Dorsal Vagal Complex and Its Resident Preproglucagon Neurons.
Holt MK, Pomeranz LE, Beier KT, Reimann F, Gribble FM, Rinaman L. Holt MK, et al. J Neurosci. 2019 Dec 4;39(49):9767-9781. doi: 10.1523/JNEUROSCI.2145-19.2019. Epub 2019 Oct 30. J Neurosci. 2019. PMID: 31666353 Free PMC article.
-
Rinaman L. Rinaman L. J Neurosci. 2003 Nov 5;23(31):10084-92. doi: 10.1523/JNEUROSCI.23-31-10084.2003. J Neurosci. 2003. PMID: 14602823 Free PMC article.
-
Peptide signals regulating food intake and energy homeostasis.
Blevins JE, Schwartz MW, Baskin DG. Blevins JE, et al. Can J Physiol Pharmacol. 2002 May;80(5):396-406. doi: 10.1139/y02-035. Can J Physiol Pharmacol. 2002. PMID: 12056545 Review.
Cited by
-
Vagal afferent controls of feeding: a possible role for gastrointestinal BDNF.
Fox EA. Fox EA. Clin Auton Res. 2013 Feb;23(1):15-31. doi: 10.1007/s10286-012-0170-x. Epub 2012 Jun 21. Clin Auton Res. 2013. PMID: 22717678 Review.
-
Maniscalco JW, Zheng H, Gordon PJ, Rinaman L. Maniscalco JW, et al. J Neurosci. 2015 Jul 29;35(30):10701-14. doi: 10.1523/JNEUROSCI.3464-14.2015. J Neurosci. 2015. PMID: 26224855 Free PMC article.
-
Grill HJ, Hayes MR. Grill HJ, et al. Cell Metab. 2012 Sep 5;16(3):296-309. doi: 10.1016/j.cmet.2012.06.015. Epub 2012 Aug 16. Cell Metab. 2012. PMID: 22902836 Free PMC article. Review.
-
Allingbjerg ML, Hansen SN, Secher A, Thomsen M. Allingbjerg ML, et al. Exp Clin Psychopharmacol. 2023 Jun;31(3):612-620. doi: 10.1037/pha0000620. Epub 2022 Dec 8. Exp Clin Psychopharmacol. 2023. PMID: 36480394 Free PMC article.
-
Merkel R, Moreno A, Zhang Y, Herman R, Ben Nathan J, Zeb S, Rahematpura S, Stecyk K, Milliken BT, Hayes MR, Doyle RP, Schmidt HD. Merkel R, et al. Neurosci Biobehav Rev. 2021 Dec;131:1169-1179. doi: 10.1016/j.neubiorev.2021.10.026. Epub 2021 Oct 29. Neurosci Biobehav Rev. 2021. PMID: 34715149 Free PMC article. Review.
References
-
- Al-Damluji S. Adrenergic mechanisms in the control of corticotropin secretion. Journal of Endocrinology. 1988;119:5–14. - PubMed
-
- Alonso G, Szafarczyk A, Balmefrezol M, Assenmacher I. Immunocytochemical evidence of stimulatory control by the ventral noradrenergic bundle of parvicellular neurons of the paraventricular nucleus secreting corticotropin-releasing hormone and vasopressin in rats. Brain Research. 1986;397:297–307. - PubMed
-
- Altschuler SM, Rinaman L, Miselis RR. Viscerotopic representation of the alimentary tract in the dorsal and ventral vagal complex in the rat. In: Ritter S, Ritter RC, Barnes CD, editors. Neuroanatomy and Physiology of Abdominal Vagal Afferents. Vol. Boca Raton: CRC Press; 1992. pp. 21–54.
-
- Altschuler SM, Bao X, Bieger D, Hopkins DA, Miselis RR. Viscerotopic representation of the upper alimentary tract in the rat: sensory ganglia and nuclei of the solitary and spinal trigeminal tracts. Journal of Comparative Neurology. 1989;283:248–268. - PubMed
Publication types
MeSH terms
Substances
Grants and funding
LinkOut - more resources
Full Text Sources