Temperature dependence of protein motions in a thermophilic dihydrofolate reductase and its relationship to catalytic efficiency - PubMed
- ️Fri Jan 01 2010
Temperature dependence of protein motions in a thermophilic dihydrofolate reductase and its relationship to catalytic efficiency
Olayinka A Oyeyemi et al. Proc Natl Acad Sci U S A. 2010.
Abstract
We report hydrogen deuterium exchange by mass spectrometry (HDX-MS) as a function of temperature in a thermophilic dihydrofolate reductase from Bacillus stearothermophilus (Bs-DHFR). Protein stability, probed with circular dichroism, established an accessible temperature range of 10 degrees C to 55 degrees C for the interrogation of HDX-MS. Although both the rate and extent of HDX are sensitive to temperature, the majority of peptides showed rapid kinetics of exchange, allowing us to focus on plateau values for the maximal extent of exchange at each temperature. Arrhenius plots of the ratio of hydrogens exchanged at 5 h normalized to the number of exchangeable hydrogens vs. 1/T provides an estimate for the apparent enthalpic change of local unfolding, DeltaH degrees (unf(avg)). Most regions in the enzyme show DeltaH degrees (unf(avg)) < or = 2.0 kcal/mol, close to the value of kT; by contrast, significantly elevated values for DeltaH degrees (unf(avg)) are observed in regions within the core of protein that contain the cofactor and substrate-binding sites. Our technique introduces a new strategy for probing the temperature dependence of local protein unfolding within native proteins. These findings are discussed in the context of the demonstrated role for nuclear tunneling in hydride transfer from NADPH to dihydrofolate, and relate the observed enthalpic changes to two classes of motion, preorganization and reorganization, that have been proposed to control the efficiency of hydrogenic wave function overlap. Our findings suggest that the enthalpic contribution to the heavy atom environmental reorganizations controlling the hydrogenic wave function overlap will be dominated by regions of the protein proximal to the bound cofactor and substrate.
Conflict of interest statement
The authors declare no conflict of interest.
Figures
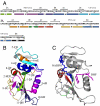
Peptic fragments of Bs-DHFR. (A) Eleven nonoverlapping fragments of Bs-DHFR generated after proteolysis by pepsin at pH 2.4. (B) The pepsin-generated fragments are mapped onto the X-ray structure of Bs-DHFR (PDBID:1ZDR), according to colors in (A). (C) X-ray structure of Ec-DHFR, indicating bound dihydrofolate (DHF) (rose) and cofactor NADPH (blue).
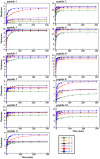
HDX time courses of Bs-DHFR peptides as a function of temperature. Extent of deuteration in peptides of Bs-DHFR, after incubating protein in D2O for 10 s–5 h at 10 (green), 25 (orange), 35 (black), 40 (purple), 50 (red), and 55 (blue). The top of each graph indicates the maximal number of exchangeable amides corrected to 100% D2O.
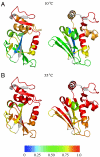
Regional variations in HDX temperature effects. (A) HDX at t = 300 min and 10 °C shows high solvent exchange in the adenosine-binding subdomain and αB of Bs-DHFR. In contrast, most of the loop subdomain including M20, FG and GH loops shows intermediate accessibility to solvent, and there is strong protection from exchange in the protein core (βA and βF). (B) At 55 °C, deuteration increases, reaching maximal levels in parts of the loop subdomain, although still submaximal in the M20, FG, and GH loops. The largest temperature dependence of deuteration occurs in βA and αF.
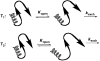
Schematic illustrating the temperature-dependent disruption of local secondary structures within a native folded protein. According to this model, temperature affects not only the equilibrium constant, Kopen, but also the structure of the opened state of protein. T1 is the lowest initial temperature and T2, an elevated temperature.
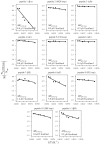
Regional variations in the enthalpy of HDX. Plots of ln (Nt,t=300 min/N∞) vs. 1/T show the linear dependence, with indicated in the frame of each peptide.
Similar articles
-
Oyeyemi OA, Sours KM, Lee T, Kohen A, Resing KA, Ahn NG, Klinman JP. Oyeyemi OA, et al. Biochemistry. 2011 Sep 27;50(38):8251-60. doi: 10.1021/bi200640s. Epub 2011 Aug 30. Biochemistry. 2011. PMID: 21859100 Free PMC article.
-
Miyagi M, Wan Q, Ahmad MF, Gokulrangan G, Tomechko SE, Bennett B, Dealwis C. Miyagi M, et al. PLoS One. 2011 Feb 16;6(2):e17055. doi: 10.1371/journal.pone.0017055. PLoS One. 2011. PMID: 21359214 Free PMC article.
-
Distal Regions Regulate Dihydrofolate Reductase-Ligand Interactions.
Goldstein M, Goodey NM. Goldstein M, et al. Methods Mol Biol. 2021;2253:185-219. doi: 10.1007/978-1-0716-1154-8_12. Methods Mol Biol. 2021. PMID: 33315225 Review.
-
Hydrogen tunneling and protein motion in enzyme reactions.
Hammes-Schiffer S. Hammes-Schiffer S. Acc Chem Res. 2006 Feb;39(2):93-100. doi: 10.1021/ar040199a. Acc Chem Res. 2006. PMID: 16489728 Review.
Cited by
-
Lisi GP, Currier AA, Loria JP. Lisi GP, et al. Front Mol Biosci. 2018 Feb 6;5:4. doi: 10.3389/fmolb.2018.00004. eCollection 2018. Front Mol Biosci. 2018. PMID: 29468164 Free PMC article.
-
Update 1 of: Tunneling and dynamics in enzymatic hydride transfer.
Nagel ZD, Klinman JP. Nagel ZD, et al. Chem Rev. 2010 Dec 8;110(12):PR41-67. doi: 10.1021/cr1001035. Chem Rev. 2010. PMID: 21141912 Free PMC article. No abstract available.
-
Wilderman PR, Shah MB, Liu T, Li S, Hsu S, Roberts AG, Goodlett DR, Zhang Q, Woods VL Jr, Stout CD, Halpert JR. Wilderman PR, et al. J Biol Chem. 2010 Dec 3;285(49):38602-11. doi: 10.1074/jbc.M110.180646. Epub 2010 Sep 28. J Biol Chem. 2010. PMID: 20880847 Free PMC article.
-
Offenbacher AR, Hu S, Poss EM, Carr CAM, Scouras AD, Prigozhin DM, Iavarone AT, Palla A, Alber T, Fraser JS, Klinman JP. Offenbacher AR, et al. ACS Cent Sci. 2017 Jun 28;3(6):570-579. doi: 10.1021/acscentsci.7b00142. Epub 2017 Jun 9. ACS Cent Sci. 2017. PMID: 28691068 Free PMC article.
-
Hydrogen tunneling links protein dynamics to enzyme catalysis.
Klinman JP, Kohen A. Klinman JP, et al. Annu Rev Biochem. 2013;82:471-96. doi: 10.1146/annurev-biochem-051710-133623. Annu Rev Biochem. 2013. PMID: 23746260 Free PMC article. Review.
References
-
- Blakley R. In: Folates and Pterins. Blakley RA, Benkovic SJ, editors. New York: Wiley; 1984. pp. 191–253.
-
- Peppard WJ, Schuenke CD. Iclaprim, a diaminopyrimidine dihydrofolate reductase inhibitor for the potential treatment of antibiotic-resistant staphylococcal infections. Curr Opin Invest Dr. 2008;9:210–225. - PubMed
-
- Gangjee A, Kurup S, Namjoshi O. Dihydrofolate reductase as a target for chemotherapy in parasites. Curr Pharm Design. 2007;13:609–639. - PubMed
-
- Soeiro MNC, de Castro SL. Trypanosoma cruzi targets for new chemotherapeutic approaches. Expert Opin Ther Tar. 2009;13:105–121. - PubMed
-
- Sikorski RS, et al. Tunneling and coupled motion in the E. coli dihydrofolate reductase catalysis. J Am Chem Soc. 2004;126:4778–4779. - PubMed
Publication types
MeSH terms
Substances
Grants and funding
LinkOut - more resources
Full Text Sources
Other Literature Sources