Altered manganese homeostasis and manganese toxicity in a Huntington's disease striatal cell model are not explained by defects in the iron transport system - PubMed
Altered manganese homeostasis and manganese toxicity in a Huntington's disease striatal cell model are not explained by defects in the iron transport system
B Blairanne Williams et al. Toxicol Sci. 2010 Sep.
Abstract
Expansion of a polyglutamine tract in Huntingtin (Htt) leads to the degeneration of medium spiny neurons in Huntington's disease (HD). Furthermore, the HTT gene has been functionally linked to iron (Fe) metabolism, and HD patients show alterations in brain and peripheral Fe homeostasis. Recently, we discovered that expression of mutant HTT is associated with impaired manganese (Mn) uptake following overexposure in a striatal neuronal cell line and mouse model of HD. Here we test the hypothesis that the transferrin receptor (TfR)-mediated Fe uptake pathway is responsible for the HD-associated defects in Mn uptake. Western blot analysis showed that TfR levels are reduced in the mutant STHdh(Q111/Q111) striatal cell line, whereas levels of the Fe and Mn transporter, divalent metal transporter 1 (DMT1), are unchanged. To stress the Fe transport system, we exposed mutant and wild-type cells to elevated Fe(III), which revealed a subtle impairment in net Fe uptake only at the highest Fe exposures. In contrast, the HD mutant line exhibited substantial deficits in net Mn uptake, even under basal conditions. Finally, to functionally evaluate a role for Fe transporters in the Mn uptake deficit, we examined Mn toxicity in the presence of saturating Fe(III) levels. Although Fe(III) exposure decreased Mn neurotoxicity, it did so equally for wild-type and mutant cells. Therefore, although Fe transporters contribute to Mn uptake and toxicity in the striatal cell lines, functional alterations in this pathway are insufficient to explain the strong Mn resistance phenotype of this HD cell model.
Figures
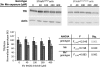
Decrease levels of total TfR in HD striatal cells. Lysates harvested from STHdhQ7/Q7 (dark gray bars) or STHdhQ111/Q111 (light gray bars) cell lines after 3 h of the indicated level of Mn(II) chloride exposure and vehicle control were analyzed by Western blot for TfR and actin protein levels. Quantification of TfR protein expression in striatal cell lines, bar graph plotted as mean levels (±SEM) normalized to actin as a percent of the untreated wild-type control (n = 4 independent samples). Table shows statistical analysis of a two-factor univariate ANOVA for relative TfR protein levels, a significant effect of genotype is designated by shaded numbering.
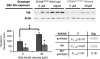
Diminished response of Fe pathway to Mn in HD model. Lysates harvested from STHdhQ7/Q7 (dark gray bars) or STHdhQ111/Q111 (light gray bars) cell lines after 30 h of Mn(II) chloride exposure versus vehicle control were analyzed by Western blot for TfR and actin. Representative blots showing three samples of each genotype-exposure group are shown, Mn(II) exposure as indicated. Quantification of TfR protein expression in striatal cell lines, bar graph plotted as mean levels (±SEM) normalized to actin as a percent of the untreated wild-type control (n = 7). Table shows statistical analysis of a two-factor univariate ANOVA for relative TfR protein levels, significant effects of genotype and Mn(II) exposure are designated by shaded numbering. “*” Indicates significant differences between the four genotype-treatment groups (p < 0.05, post hoc t-test).
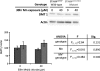
DMT1 levels unchanged in HD striatal cells. Lysates harvested from STHdhQ7/Q7 (dark gray bars) or STHdhQ111/Q111 (light gray bars) cell lines after 30 h of Mn(II) chloride exposure versus vehicle control were analyzed by Western blot for TfR and actin. Representative blot showing one set of samples analyzed, Mn(II) exposure as indicated. Quantification of TfR protein expression in striatal cell lines, bar graph plotted as mean levels (±SEM) normalized to actin as a percent of the untreated wild-type control (n = 3 independent samples). Table shows statistical analysis of a two-factor univariate ANOVA for relative DMT1 protein levels, no significant effects were observed, though genotype exhibited a trend toward increased DMT1 levels in mutant cells (p = 0.131).
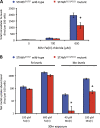
Net Fe uptake is reduced in the HD striatal cells. (A) Measurement of total intracellular Fe levels in STHdhQ7/Q7 or STHdhQ111/Q111 striatal cell lines (as indicated) after application of the indicated concentrations of Fe(II) chloride for 30 h (n = 3 to 5). Mean values (±SEM) are plotted on a linear scale. (B) Comparisons of net Fe uptake versus net Mn uptake above basal levels (vehicle control) in STHdhQ7/Q7 (blue) versus STHdhQ111/Q111 (red) cells following a 30-h exposure to these metals. Calculations are derived from Fe data in (Fig. 4A) and previously published Mn data (Williams et al., 2010). Data expressed as the absolute difference (±SEM) between metal-exposed and vehicle-exposed cells (n = 3–5), plotted on log10 scale. “*” Indicates a significant difference in net metal uptake (p < 0.05, using 95% confidence intervals) between wild-type and mutant cells.
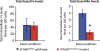
Basal Mn deficiency in HD striatal cells. Measurement of total intracellular Fe and Mn by GFAAS of cells collected from two 10-cm2 plates per sample of STHdhQ7/Q7 or STHdhQ111/Q111 cells (as indicated). Cells grown under standard culture conditions without supplemented Mn(II) or Fe(III). The mean total metal levels (±SEM) are plotted as indicated. “*” Indicates a significant difference in measured metal levels (p < 0.05, t-test) between wild-type and mutant cells.
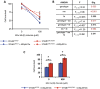
Saturating levels of Fe have no significant effect on the HTT-Mn interaction. Wild-type STHdhQ7/Q7 and mutant STHdhQ111/Q111 cells (as indicated) were exposed for 30 h to Mn(II) chloride in the presence (dashed line) or absence (solid line) of 600μM Fe(III) chloride. (A) Cell survival by MTT assay was determined (n = 3 independent experiments, 4 wells per experiment) and graphed as mean survival (±SEM) normalized by the vehicle control (no Mn or Fe) for each genotype. (B) Table summarizes statistical analysis of three-way repeated measures ANOVA (genotype, Mn(II) exposure, Fe(III) exposure, and all two-way and three-way interaction terms); significant terms (p < 0.05) are indicated by shaded numbering. (C) Cell survival data for 100μM Mn(II) chloride exposure only, mean survival (±SEM) normalized by genotype and Fe(III) exposure to show the relative influence of Fe on Mn toxicity between wild-type and mutant cells (as indicated). Mutant cells show a significant increase in survival following Mn exposure both with and without Fe exposure. Note that the magnitude of the difference between genotypes is similar, irrespective of whether there was Fe present. “*” Indicates a significant difference in survival (p < 0.05, t-test) between wild-type and mutant cells.
Similar articles
-
Kwakye GF, Li D, Bowman AB. Kwakye GF, et al. Neurotoxicology. 2011 Oct;32(5):630-9. doi: 10.1016/j.neuro.2011.01.002. Epub 2011 Jan 14. Neurotoxicology. 2011. PMID: 21238486 Free PMC article.
-
Choudhury KR, Das S, Bhattacharyya NP. Choudhury KR, et al. J Proteomics. 2016 Jan 30;132:155-66. doi: 10.1016/j.jprot.2015.11.007. Epub 2015 Nov 12. J Proteomics. 2016. PMID: 26581643
-
Zhang H, Li Q, Graham RK, Slow E, Hayden MR, Bezprozvanny I. Zhang H, et al. Neurobiol Dis. 2008 Jul;31(1):80-8. doi: 10.1016/j.nbd.2008.03.010. Epub 2008 Apr 16. Neurobiol Dis. 2008. PMID: 18502655 Free PMC article.
-
Bryan MR, Bowman AB. Bryan MR, et al. Adv Neurobiol. 2017;18:113-142. doi: 10.1007/978-3-319-60189-2_6. Adv Neurobiol. 2017. PMID: 28889265 Free PMC article. Review.
-
Selective degeneration in YAC mouse models of Huntington disease.
Van Raamsdonk JM, Warby SC, Hayden MR. Van Raamsdonk JM, et al. Brain Res Bull. 2007 Apr 30;72(2-3):124-31. doi: 10.1016/j.brainresbull.2006.10.018. Epub 2006 Nov 16. Brain Res Bull. 2007. PMID: 17352936 Review.
Cited by
-
Castiglione A, Ciorba A, Aimoni C, Orioli E, Zeri G, Vigliano M, Gemmati D. Castiglione A, et al. Biomed Res Int. 2015;2015:834736. doi: 10.1155/2015/834736. Epub 2015 Feb 18. Biomed Res Int. 2015. PMID: 25789325 Free PMC article.
-
Joshi P, Bodnya C, Ilieva I, Neely MD, Aschner M, Bowman AB. Joshi P, et al. Neurotoxicology. 2019 Dec;75:148-157. doi: 10.1016/j.neuro.2019.09.007. Epub 2019 Sep 20. Neurotoxicology. 2019. PMID: 31545971 Free PMC article.
-
Amos-Kroohs RM, Bloor CP, Qureshi MA, Vorhees CV, Williams MT. Amos-Kroohs RM, et al. Toxicol Rep. 2015;2:1046-1056. doi: 10.1016/j.toxrep.2015.07.015. Toxicol Rep. 2015. PMID: 26295019 Free PMC article.
-
Kumar KK, Goodwin CR, Uhouse MA, Bornhorst J, Schwerdtle T, Aschner M, McLean JA, Bowman AB. Kumar KK, et al. Metallomics. 2015 Feb;7(2):363-70. doi: 10.1039/c4mt00223g. Metallomics. 2015. PMID: 25599126 Free PMC article.
-
Mechanisms of Metal-Induced Mitochondrial Dysfunction in Neurological Disorders.
Cheng H, Yang B, Ke T, Li S, Yang X, Aschner M, Chen P. Cheng H, et al. Toxics. 2021 Jun 17;9(6):142. doi: 10.3390/toxics9060142. Toxics. 2021. PMID: 34204190 Free PMC article. Review.
References
-
- Anderson J, Fordahl S, Cooney P, Weaver T, Colyer C, Erikson K. Extracellular norepinephrine, norepinephrine receptor and transporter protein and mRNA levels are differentially altered in the developing rat brain due to dietary iron deficiency and manganese exposure. Brain Res. 2009;1281:1–14. - PMC - PubMed
-
- Andreassen O, Ferrante R, Dedeoglu A, Albers D, Klivenyi P, Carlson E, Epstein C, Beal M. Mice with a partial deficiency of manganese superoxide dismutase show increased vulnerability to the mitochondrial toxins malonate, 3-nitropropionic acid, and MPTP. Exp. Neurol. 2001;167:189–195. - PubMed
-
- Applebury M, Johnson B, Coleman J. Phosphate binding to alkaline phosphatase. Metal ion dependence. J. Biol. Chem. 1970;245:4968–4976. - PubMed
-
- Aschner M, Erikson K, Dorman D. Manganese dosimetry: species differences and implications for neurotoxicity. Crit. Rev. Toxicol. 2005;35:1–32. - PubMed
-
- Aschner M, Gannon M. Manganese (Mn) transport across the rat blood-brain barrier: saturable and transferrin-dependent transport mechanisms. Brain Res. Bull. 1994;33:345–349. - PubMed
Publication types
MeSH terms
Substances
Grants and funding
LinkOut - more resources
Full Text Sources
Medical