Repetitive antigen stimulation induces stepwise transcriptome diversification but preserves a core signature of memory CD8(+) T cell differentiation - PubMed
- ️Fri Jan 01 2010
Repetitive antigen stimulation induces stepwise transcriptome diversification but preserves a core signature of memory CD8(+) T cell differentiation
Thomas C Wirth et al. Immunity. 2010.
Abstract
Repetitive antigen stimulation by prime-boost vaccination or pathogen reencounter increases memory CD8(+) T cell numbers, but the impact on memory CD8(+) T cell differentiation is unknown. Here we showed that repetitive antigen stimulations induced accumulation of memory CD8(+) T cells with uniform effector memory characteristics. However, genome-wide microarray analyses revealed that each additional antigen challenge resulted in the differential regulation of several hundred new genes in the ensuing memory CD8(+) T cell populations and, therefore, in stepwise diversification of CD8(+) T cell transcriptomes. Thus, primary and repeatedly stimulated (secondary, tertiary, and quaternary) memory CD8(+) T cells differed substantially in their molecular signature while sharing expression of a small group of genes and biological pathways, which may constitute a core signature of memory differentiation. These results reveal the complex regulation of memory CD8(+) T cell differentiation and identify potential new molecular targets to dissect the function of memory cells generated by repeated antigen stimulation.
Copyright 2010 Elsevier Inc. All rights reserved.
Figures
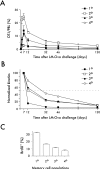
Naïve (5×102) or memory (6×104) Thy1.1 OT-I T cells were adoptively transferred into naïve Thy1.2 C57Bl/6 recipient mice prior to infection with 5×106 att LM-OVA. A) OT-I T cell responses (% of OT-I T cells of all PBL) in peripheral blood. Data are mean +/− SD for 3–10 mice per group per time point. B) Percentages of OT-I T cells in PBL were normalized to the expansion peak at day 7 for each individual group. Data are mean +/− SD for 3–10 mice per group per time point. C) Proliferation of memory cells was determined by BrdU incorporation (day 70–84). Bar graphs represent the percentage of BrdU+ OT-I T cells in spleen (mean +/− SD, n=5).
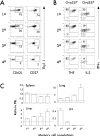
A) Phenotype of OT-I T cells in the spleen 90 days post infection. Numbers inside dot plots represent the percentage of cells positive for the indicated molecules. B) Peptide-stimulated intracellular cytokine staining (ICS) of OT-I T cells from spleen. Numbers represent the percentage of OT-I T cells that stain positive for a given cytokine combination. C) OT-I T cell frequency in total CD8+ T cells in tissues was normalized to the frequency in PBL. Data are mean +/− SD for 3 mice per group.
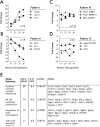
Genome-wide mRNA expression in memory OT-I T cells was analyzed via microarray and normalized to naive OT-I T cells. An FDR q-value <0.01 defined significant changes. Genes with significant mRNA changes in all memory OT-I T cell populations (1° to 4°) were grouped according to gene expression patterns: A) transcriptional upregulation with consistent increase (pattern I), B) transcriptional downregulation with consistent decrease (pattern II), C) transcriptional upregulation with no consistent increase (pattern III), or D) transcriptional downregulation with no consistent decrease (pattern IV) from 1° to 4° memory. E) Biological pathway analysis of genes with significant mRNA changes in all memory CD8+ T cell populations was generated using KEGG pathway tool in DAVID bioinformatics resources. Arrows indicate whether genes are upregulated (↑) or downregulated (↓) in all memory CD8+ T cell populations when compared to naïve cells.
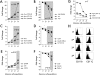
Genes with the following expression patterns were identified: A) transcriptional upregulation or B) downregulation in 2°, 3° and 4° memory, C) transcriptional upregulation or D) downregulation in 3° and 4° memory, E) transcriptional upregulation or F) downregulation in 4° memory, G) transcriptional upregulation in 1° memory followed by decreasing mRNA expression from 1° to 4° memory. H) Protein expression of CD11b and CD11c on memory CD8+ T cells 90 days after infection (see Figure 3A+G for mRNA expression). Representative histograms are shown.
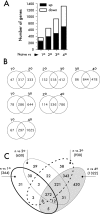
A) Genome-wide gene transcription in 1°, 2°, 3°, and 4° memory OT-I T cell (day 85–95 p.i.) populations was analyzed via microarray and compared to naïve OT-I T cells. An FDR q-value <= 0.01 defined statistically significance. Data represent the number of genes with significant regulation inside each group. B) Venn diagram presentation of genes with significant mRNA changes in indicated memory CD8+ T cell populations. Overlapping circles represent number of genes whose expression is different from naïve cells but is either shared or unique to indicated memory CD8+ T cell groups. C) Significantly altered genes were assessed for all four memory groups individually. Each memory group is represented by an individual ellipse, overlapping ellipses indicate genes shared by two or more groups.
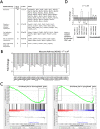
A) Genes with significant mRNA changes between 1° and 4° memory CD8 T cells (Table S4) were evaluated using KEGG pathway analysis in DAVID. Arrows indicate whether genes are upregulated (↑) or downregulated (↓) in 4° compared to 1° memory CD8 T cells. B) Fold changes in mRNA of ribosomal genes in 4° compared to 1° memory CD8+ T cells. C) GSEA was performed to compare whether exhaustion-associated genes (down (left panel) or up (right panel)) showed specific enrichment in 4° memory CD8+ T cells. Rectangles with solid lines indicate down- or up-regulated genes common to exhausted and 4° memory CD8+ T cells. Rectangles with dotted lines indicate genes showing opposite expression patterns between exhausted and 4° memory CD8+ T cells. Genes are shown in Table S5. D) Fold changes in mRNA quantity for selected inhibitory receptors (implicated in exhausted CD8+ T cells (Shin et al., 2009)) in 4° compared to 1° memory CD8+ T cells.
Similar articles
-
Modulating numbers and phenotype of CD8+ T cells in secondary immune responses.
Wirth TC, Harty JT, Badovinac VP. Wirth TC, et al. Eur J Immunol. 2010 Jul;40(7):1916-26. doi: 10.1002/eji.201040310. Eur J Immunol. 2010. PMID: 20411564 Free PMC article.
-
Exploiting cross-priming to generate protective CD8 T-cell immunity rapidly.
Pham NL, Pewe LL, Fleenor CJ, Langlois RA, Legge KL, Badovinac VP, Harty JT. Pham NL, et al. Proc Natl Acad Sci U S A. 2010 Jul 6;107(27):12198-203. doi: 10.1073/pnas.1004661107. Epub 2010 Jun 21. Proc Natl Acad Sci U S A. 2010. PMID: 20616089 Free PMC article.
-
Pedicord VA, Cross JR, Montalvo-Ortiz W, Miller ML, Allison JP. Pedicord VA, et al. J Immunol. 2015 Mar 1;194(5):2089-98. doi: 10.4049/jimmunol.1402390. Epub 2015 Jan 26. J Immunol. 2015. PMID: 25624453
-
Influence of time and number of antigen encounters on memory CD8 T cell development.
Martin MD, Badovinac VP. Martin MD, et al. Immunol Res. 2014 Aug;59(1-3):35-44. doi: 10.1007/s12026-014-8522-3. Immunol Res. 2014. PMID: 24825776 Review.
-
Signals for antigen-independent differentiation of memory CD8+ T cells.
Kwesi-Maliepaard EM, Jacobs H, van Leeuwen F. Kwesi-Maliepaard EM, et al. Cell Mol Life Sci. 2021 Oct;78(19-20):6395-6408. doi: 10.1007/s00018-021-03912-9. Epub 2021 Aug 16. Cell Mol Life Sci. 2021. PMID: 34398252 Free PMC article. Review.
Cited by
-
Xia J, Kuang Y, Liang J, Jones M, Swain SL. Xia J, et al. J Immunol. 2020 Oct 15;205(8):2077-2090. doi: 10.4049/jimmunol.2000597. Epub 2020 Sep 14. J Immunol. 2020. PMID: 32929040 Free PMC article.
-
BACH2 regulates CD8(+) T cell differentiation by controlling access of AP-1 factors to enhancers.
Roychoudhuri R, Clever D, Li P, Wakabayashi Y, Quinn KM, Klebanoff CA, Ji Y, Sukumar M, Eil RL, Yu Z, Spolski R, Palmer DC, Pan JH, Patel SJ, Macallan DC, Fabozzi G, Shih HY, Kanno Y, Muto A, Zhu J, Gattinoni L, O'Shea JJ, Okkenhaug K, Igarashi K, Leonard WJ, Restifo NP. Roychoudhuri R, et al. Nat Immunol. 2016 Jul;17(7):851-860. doi: 10.1038/ni.3441. Epub 2016 May 9. Nat Immunol. 2016. PMID: 27158840 Free PMC article.
-
Chung HK, McDonald B, Kaech SM. Chung HK, et al. J Exp Med. 2021 Apr 5;218(4):e20201730. doi: 10.1084/jem.20201730. J Exp Med. 2021. PMID: 33755719 Free PMC article. Review.
-
Th17 cells are long lived and retain a stem cell-like molecular signature.
Muranski P, Borman ZA, Kerkar SP, Klebanoff CA, Ji Y, Sanchez-Perez L, Sukumar M, Reger RN, Yu Z, Kern SJ, Roychoudhuri R, Ferreyra GA, Shen W, Durum SK, Feigenbaum L, Palmer DC, Antony PA, Chan CC, Laurence A, Danner RL, Gattinoni L, Restifo NP. Muranski P, et al. Immunity. 2011 Dec 23;35(6):972-85. doi: 10.1016/j.immuni.2011.09.019. Epub 2011 Dec 15. Immunity. 2011. PMID: 22177921 Free PMC article.
-
Stai S, Lioulios G, Xochelli A, Papadopoulou A, Yannaki E, Kasimatis E, Christodoulou M, Moysidou E, Samali M, Testa T, Iosifidou AM, Iosifidou MA, Tsoulfas G, Stangou M, Fylaktou A. Stai S, et al. Vaccines (Basel). 2024 Aug 2;12(8):877. doi: 10.3390/vaccines12080877. Vaccines (Basel). 2024. PMID: 39204004 Free PMC article.
References
-
- Appay V, Douek DC, Price DA. CD8 T cell efficacy in vaccination and disease. Nat Med. 2008;14:623–628. - PubMed
-
- Badovinac VP, Messingham KA, Hamilton SE, Harty JT. Regulation of CD8 T cells undergoing primary and secondary responses to infection in the same host. J Immunol. 2003;170:4933–4942. - PubMed
-
- Badovinac VP, Messingham KA, Jabbari A, Haring JS, Harty JT. Accelerated CD8 T-cell memory and prime-boost response after dendritic-cell vaccination. Nat Med. 2005;11:748–756. - PubMed
-
- Badovinac VP, Porter BB, Harty JT. Programmed contraction of CD8 T cells after infection. Nat Immunol. 2002;3:619–626. - PubMed
Publication types
MeSH terms
Substances
Grants and funding
- R01 AI050073/AI/NIAID NIH HHS/United States
- R01 AI083286/AI/NIAID NIH HHS/United States
- R37 AI042767-10/AI/NIAID NIH HHS/United States
- AI59752/AI/NIAID NIH HHS/United States
- R01 AI042767/AI/NIAID NIH HHS/United States
- AI50073/AI/NIAID NIH HHS/United States
- R01 AI050073-10/AI/NIAID NIH HHS/United States
- R21 AI077504/AI/NIAID NIH HHS/United States
- R01 AI046653/AI/NIAID NIH HHS/United States
- R37 AI042767/AI/NIAID NIH HHS/United States
- HL095540/HL/NHLBI NIH HHS/United States
- R01 AI059752-05/AI/NIAID NIH HHS/United States
- R01 AI046653-09/AI/NIAID NIH HHS/United States
- R01 AI059752/AI/NIAID NIH HHS/United States
- AI077504/AI/NIAID NIH HHS/United States
- AI83286/AI/NIAID NIH HHS/United States
- AI42767/AI/NIAID NIH HHS/United States
- R01 HL095540/HL/NHLBI NIH HHS/United States
- R21 AI042767/AI/NIAID NIH HHS/United States
- AI46653/AI/NIAID NIH HHS/United States
LinkOut - more resources
Full Text Sources
Other Literature Sources
Medical
Molecular Biology Databases
Research Materials