Overcoming the heme paradox: heme toxicity and tolerance in bacterial pathogens - PubMed
Review
Overcoming the heme paradox: heme toxicity and tolerance in bacterial pathogens
Laura L Anzaldi et al. Infect Immun. 2010 Dec.
Abstract
Virtually all bacterial pathogens require iron to infect vertebrates. The most abundant source of iron within vertebrates is in the form of heme as a cofactor of hemoproteins. Many bacterial pathogens have elegant systems dedicated to the acquisition of heme from host hemoproteins. Once internalized, heme is either degraded to release free iron or used intact as a cofactor in catalases, cytochromes, and other bacterial hemoproteins. Paradoxically, the high redox potential of heme makes it a liability, as heme is toxic at high concentrations. Although a variety of mechanisms have been proposed to explain heme toxicity, the mechanisms by which heme kills bacteria are not well understood. Nonetheless, bacteria employ various strategies to protect against and eliminate heme toxicity. Factors involved in heme acquisition and detoxification have been found to contribute to virulence, underscoring the physiological relevance of heme stress during pathogenesis. Herein we describe the current understanding of the mechanisms of heme toxicity and how bacterial pathogens overcome the heme paradox during infection.
Figures
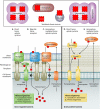
Mechanisms of bacterial heme acquisition. Bacteria can utilize multiple heme sources found within the vertebrate host. Three types of heme acquisition systems have been identified for Gram-negative bacteria (left). These systems include direct heme uptake systems (A), bipartite heme receptors (B), and hemophore-mediated heme uptake systems (C). Most Gram-negative heme acquisition systems rely on an energy-transducing protein such as TonB to transport heme across the OM. Typically, Gram-positive bacteria (right) use direct heme uptake systems (D) to acquire heme. Recently, the first Gram-positive hemophore-mediated heme acquisition system (E) was identified in Bacillus anthracis. Heme is represented by the red polygons.
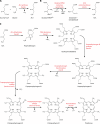
Schematic of heme biosynthesis in bacteria. The first universal heme precursor is δ-aminolevulinic acid (ALA). ALA can be synthesized by one of two routes, although no bacteria are known to use both ALA synthesis pathways. (A) Succinyl-CoA and glycine are converted into ALA by ALA synthase (hemA). HemA, however, has been identified only in members of the Alphaproteobacteria. (B) Most bacteria synthesize ALA from glutamyl-tRNAGlu by the C5 pathway using GtrA and HemL. (C) The rest of the heme biosynthesis pathway is highly conserved. ALA dehydratase (hemB) synthesizes porphobilinogen (PBG) from two ALA molecules. PBG deaminase (hemC) converts four PBG molecules into hydroxymethylbilane. Linear hydroxymethylbilane is fused into a ring by uroporphyrinogen III synthase (hemD) to make uroporphyrinogen III. Four carboxyl groups are removed by uroporphyrinogen decarboxylase (hemE) to make coproporphyrinogen III. Next, a coproporphyrinogen oxidase, either HemN (oxygen independent) or HemF (oxygen dependent), converts coproporphyrinogen III to protoporphyrinogen IX. Protoporphyrinogen IX is then oxidized to protoporphyrin IX by a protoporphyrinogen oxidase, either HemG (O2 independent) or HemY (O2 dependent) (8, 38). Finally, ferrochelatase (hemH) catalyzes the protoporphyrin IX chelation of ferrous iron to form protoheme. This protoheme can be utilized directly or modified further before being used as a prosthetic group in hemoproteins.
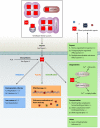
Overview of the heme paradox in bacteria. Most Gram-negative and Gram-positive bacteria are capable of acquiring or synthesizing heme. Heme can then be used for cellular processes (blue boxes). However, an accumulation of intracellular heme can cause toxicity to the cell (orange boxes). Bacteria utilize multiple mechanisms to eliminate heme toxicity (green boxes). Processes found for Gram-negative bacteria are marked with a “−” in parentheses, while those identified for Gram-positive bacteria are marked with a “+” in parentheses.
Similar articles
-
Heme Synthesis and Acquisition in Bacterial Pathogens.
Choby JE, Skaar EP. Choby JE, et al. J Mol Biol. 2016 Aug 28;428(17):3408-28. doi: 10.1016/j.jmb.2016.03.018. Epub 2016 Mar 24. J Mol Biol. 2016. PMID: 27019298 Free PMC article. Review.
-
Intracellular metalloporphyrin metabolism in Staphylococcus aureus.
Reniere ML, Torres VJ, Skaar EP. Reniere ML, et al. Biometals. 2007 Jun;20(3-4):333-45. doi: 10.1007/s10534-006-9032-0. Epub 2007 Mar 27. Biometals. 2007. PMID: 17387580 Review.
-
The role of host heme in bacterial infection.
Donegan RK. Donegan RK. Biol Chem. 2022 Oct 14;403(11-12):1017-1029. doi: 10.1515/hsz-2022-0192. Print 2022 Nov 25. Biol Chem. 2022. PMID: 36228088 Review.
-
Resonance Raman studies on the ligand-iron interactions in hemoproteins and metallo-porphyrins.
Kitagawa T, Ozaki Y, Kyogoku Y. Kitagawa T, et al. Adv Biophys. 1978;11:153-96. Adv Biophys. 1978. PMID: 27953
-
Iron uptake mechanisms as key virulence factors in bacterial fish pathogens.
Lemos ML, Balado M. Lemos ML, et al. J Appl Microbiol. 2020 Jul;129(1):104-115. doi: 10.1111/jam.14595. Epub 2020 Feb 12. J Appl Microbiol. 2020. PMID: 31994331 Review.
Cited by
-
Krüger A, Frunzke J. Krüger A, et al. Front Microbiol. 2022 Sep 7;13:997448. doi: 10.3389/fmicb.2022.997448. eCollection 2022. Front Microbiol. 2022. PMID: 36160252 Free PMC article.
-
Salviatto LTC, Prates RA, Pavani C, Bussadori SK, Deana AM. Salviatto LTC, et al. Lasers Med Sci. 2023 Nov 22;38(1):274. doi: 10.1007/s10103-023-03937-5. Lasers Med Sci. 2023. PMID: 37993626
-
Bacillus subtilis Fur represses one of two paralogous haem-degrading monooxygenases.
Gaballa A, Helmann JD. Gaballa A, et al. Microbiology (Reading). 2011 Nov;157(Pt 11):3221-3231. doi: 10.1099/mic.0.053579-0. Epub 2011 Aug 26. Microbiology (Reading). 2011. PMID: 21873409 Free PMC article.
-
Control of heme homeostasis in Corynebacterium glutamicum by the two-component system HrrSA.
Frunzke J, Gätgens C, Brocker M, Bott M. Frunzke J, et al. J Bacteriol. 2011 Mar;193(5):1212-21. doi: 10.1128/JB.01130-10. Epub 2011 Jan 7. J Bacteriol. 2011. PMID: 21217007 Free PMC article.
-
Benson MA, Lilo S, Wasserman GA, Thoendel M, Smith A, Horswill AR, Fraser J, Novick RP, Shopsin B, Torres VJ. Benson MA, et al. Mol Microbiol. 2011 Aug;81(3):659-75. doi: 10.1111/j.1365-2958.2011.07720.x. Epub 2011 Jun 16. Mol Microbiol. 2011. PMID: 21651625 Free PMC article.
References
-
- Aft, R. L., and G. C. Mueller. 1983. Hemin-mediated DNA strand scission. J. Biol. Chem. 258:12069-12072. - PubMed
-
- Aft, R. L., and G. C. Mueller. 1984. Hemin-mediated oxidative degradation of proteins. J. Biol. Chem. 259:301-305. - PubMed
-
- Arnoux, P., R. Haser, N. Izadi-Pruneyre, A. Lecroisey, and M. Czjzek. 2000. Functional aspects of the heme bound hemophore HasA by structural analysis of various crystal forms. Proteins 41:202-210. - PubMed
-
- Bibb, L. A., N. D. King, C. A. Kunkle, and M. P. Schmitt. 2005. Analysis of a heme-dependent signal transduction system in Corynebacterium diphtheriae: deletion of the chrAS genes results in heme sensitivity and diminished heme-dependent activation of the hmuO promoter. Infect. Immun. 73:7406-7412. - PMC - PubMed
Publication types
MeSH terms
Substances
Grants and funding
LinkOut - more resources
Full Text Sources
Other Literature Sources