Complementary spatial firing in place cell-interneuron pairs - PubMed
- ️Fri Jan 01 2010
Complementary spatial firing in place cell-interneuron pairs
Balázs Hangya et al. J Physiol. 2010.
Abstract
The hippocampal formation plays a pivotal role in representing the physical environment. While CA1 pyramidal cells display sharply tuned location-specific firing, the activity of many interneurons show weaker but significant spatial modulation. Although hippocampal interneurons were proposed to participate in the representation of space, their interplay with pyramidal cells in formation of spatial maps is not well understood. In this study, we investigated the spatial correlation between CA1 pyramidal cells and putative interneurons recorded concurrently in awake rats. Positively and negatively correlated pairs were found to be simultaneously present in the CA1 region. While pyramidal cell-interneuron pairs with positive spatial correlation showed similar firing maps, negative spatial correlation was often accompanied by complementary place maps, which could occur even in the presence of a monosynaptic excitation between the cells. Thus, location-specific firing increase of hippocampal interneurons is not necessarily a simple product of excitation by a pyramidal cell with a similarly positioned firing field. Based on our observation of pyramidal cells firing selectively in the low firing regions of interneurons, we speculate that the location-specific firing of place cells is partly determined by the location-specific decrease of interneuron activity that can release place cells from inhibition.
Figures
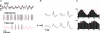
A, raw EEG trace (upper panel) shows hippocampal theta oscillation. Filtered (4–12 Hz) signal is superimposed in red. Concurrent recordings of a hippocampal interneuron (middle panel) and a place cell (lower panel) are demonstrated. Black vertical lines indicate the time instances of action potential firing. Filtered EEG trace is replicated (red) for visualization purposes. Positive spatial correlation exhibited by this pair is demonstrated in Fig. 5B. B, average action potential waveforms from the four tetrode wires. Black line, mean waveform; grey lines,
s.d.of the waveforms. Upper, interneuron; lower, place cell. C, firing phase of the neurons relative to the hippocampal theta oscillation. Idealized theta cycles are superimposed in red (two cycles are shown). The interneuron (upper) shows higher firing rates associated to the rising phase of hippocampal theta, whereas the place cell shows weak phase-locking to theta peaks.
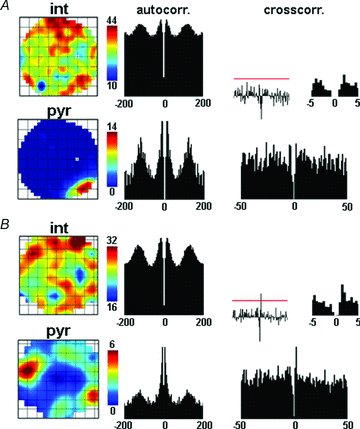
A, representative example of a place cell–interneuron pair with positively correlated place maps (R = 0.21). Arrangement of panels of Fig. 2 applies. The absence of a short latency peak in the cross-correlogram indicates that the cells are not coupled by monosynaptic excitatory connection. B, a second pyramidal cell–interneuron pair with a positive spatial correlation (R = 0.32). The significant peak of the cross-correlation within 3 ms from the centre indicates monosynaptic excitation of the interneuron by the pyramidal cell. The overlap of the high rate regions for both interneuron–pyramidal cell pairs is shown by the low complementarity indices (CI = 0 for neurons in A and B).
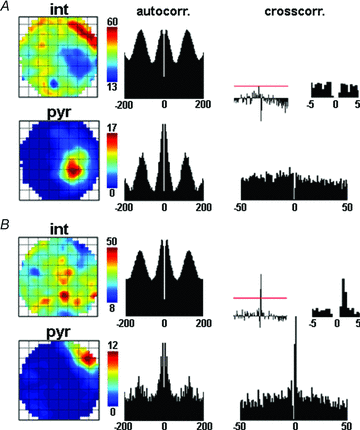
A, representative example of a pyramidal cell–interneuron pair showing negative spatial correlation (R = −0.59). Upper left, interneuron rate map (blue colour is associated to the lowest firing rate); middle, interneuron autocorrelation. Lower left, pyramidal cell rate map; middle, pyramidal cell autocorrelation. Right, cross-correlation of place cell and interneuron firing, with reference to the pyramidal cell. Left inset shows normalized cross-correlogram (see Methods); red line indicates the level of significant interaction at P = 0.0013. Right inset shows the centre of the cross-correlogram at higher temporal resolution. The absence of a significant peak within 3 ms from the centre indicates the lack of functional monosynaptic excitation of the interneuron by the pyramidal cell. B, another example of a negatively correlated pair of an interneuron and a place cell (R = −0.41). Panels are arranged as in A. The significant short latency peak in the cross-correlogram implies monosynaptic coupling of the cells. Firing field complementarity is seen for both pairs (CI = 0.66 for neurons in A, CI = 0.58 for neurons in B).
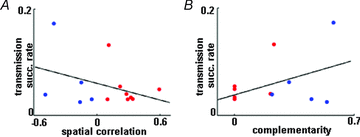
A, scatter plot showing the relationship of spatial correlation and transmission success rate for those pairs in which a monosynaptic connection between the place cell and the interneuron was established. The blue dots are for pairs with negative spatial correlation; the red dots are for pairs with positive spatial correlation. The two variables were not correlated significantly (P = 0.23). B, relationship between complementarity index and transmission success rate. The colour coding of A applies. Again, there was no significant correlation between the two parameters (P = 0.14).
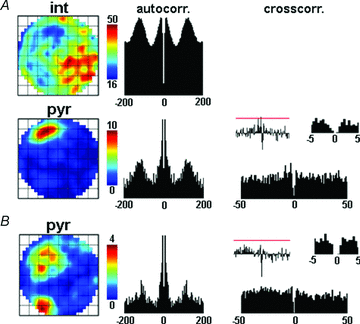
A, an interneuron–pyramidal cell pair with negative spatial correlation (R = −0.39) is presented. Panel arrangement of Fig. 2 applies also for this figure. The lack of significant short latency peak on the cross-correlogram indicates that no monosynaptic excitation between the neurons was present. B, firing characteristics of a place cell showing negative spatial correlation with the same interneuron (R = −0.41). This neuron was also not coupled to the interneuron monosynaptically. The place cell in A fired in only one of those two areas of the arena where the firing rate of the interneuron was low. In contrast, pyramidal cell in B showed two place fields corresponding to the locations characterized by low interneuron activity.
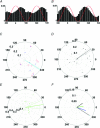
A, phase histogram of a representative interneuron participating in negatively correlated place cell–interneuron pairs (the one shown in Fig. 2A). Idealized theta cycles are superimposed in red (two cycles are shown). The interneuron is phase locked to the descending slope of the theta oscillation. B, phase histogram of the interneuron shown in Fig. 5B, participating in a positively correlated place cell–interneuron pair. Phase coupling to the rising slope of hippocampal theta is clearly visible. C, phase value distribution of each interneuron's action potential series, in which hippocampal LFP was recorded concurrently, is presented on a polar plot. In the figure, every mark represents an interneuron (blue dot, interneuron with negatively correlated place cell pair; red circle, interneuron with positively correlated pyramidal cell pair; blue dot in red circle, interneuron that participated in both type of pairs). Localization on the circle shows mean phase (zero phase represents the peak of theta oscillation), whereas distance from the centre indicates mean vector length (longer distance from centre means stronger phase coupling). The thick cyan bar represents population phase of interneurons with any type of place cell pairs; thin cyan lines indicate 95% confidence intervals of the mean. Most of the interneurons (12/13, 92%) were phase-locked to the positive phase of the theta cycle. D, phase locking of interneurons recorded from the same animals but without a detected place cell pair. The thick black bar indicates population mean and the thinner black lines in its close vicinity show 95% confidence intervals. Mean phase values occupy a domain of the unit circle that is different from that of the interneurons with correlated pairs of place cells in panel A (P < 0.001, Watson's test). Mean directions fall to the positive (9/14, 64%) or the negative phase (5/14, 36%) of hippocampal theta. E, phase locking of pyramidal neurons. Green lines indicate population mean and 95% confidence intervals. Although pyramidal neurons are coupled to the negative phase of theta oscillation in anaesthetized animals (Klausberger et al. 2003), preferred phases of principal cells recorded from freely moving rats could occupy any position on the unit circle. This difference is probably according to the modification of the preferred theta phase by the phase precession, which can result pyramidal cell firing at any particular theta phase (Schmidt et al. 2009). F, mean phase of interneurons with negatively correlated pairs (red), positively correlated pairs (blue), any type of pairs (cyan), interneurons without a detected place pair (black) and pyramidal cells (green). Lengths of the bars indicate mean vector length. Width of the cyan bar was increased to improve the visualization of the overlapping cyan and red bars. Note the different scaling of the polar plots in panels C–F.
Similar articles
-
Marshall L, Henze DA, Hirase H, Leinekugel X, Dragoi G, Buzsáki G. Marshall L, et al. J Neurosci. 2002 Jan 15;22(2):RC197. doi: 10.1523/JNEUROSCI.22-02-j0001.2002. J Neurosci. 2002. PMID: 11784809 Free PMC article.
-
Maurer AP, Cowen SL, Burke SN, Barnes CA, McNaughton BL. Maurer AP, et al. J Neurosci. 2006 Dec 27;26(52):13485-92. doi: 10.1523/JNEUROSCI.2882-06.2006. J Neurosci. 2006. PMID: 17192431 Free PMC article.
-
Bjorefeldt A, Wasling P, Zetterberg H, Hanse E. Bjorefeldt A, et al. J Physiol. 2016 Feb 15;594(4):937-52. doi: 10.1113/JP271553. Epub 2016 Jan 18. J Physiol. 2016. PMID: 26634295 Free PMC article.
-
Peng BW, Justice JA, Zhang K, He XH, Sanchez RM. Peng BW, et al. Neuropsychopharmacology. 2010 Jan;35(2):464-72. doi: 10.1038/npp.2009.150. Neuropsychopharmacology. 2010. PMID: 19776733 Free PMC article.
-
Jeong N, Singer AC. Jeong N, et al. Curr Opin Neurobiol. 2022 Oct;76:102604. doi: 10.1016/j.conb.2022.102604. Epub 2022 Jul 7. Curr Opin Neurobiol. 2022. PMID: 35810533 Free PMC article. Review.
Cited by
-
Bell LA, Bell KA, McQuiston AR. Bell LA, et al. Neuropharmacology. 2013 Oct;73:160-73. doi: 10.1016/j.neuropharm.2013.05.026. Epub 2013 Jun 5. Neuropharmacology. 2013. PMID: 23747570 Free PMC article.
-
Ran I, Laplante I, Lacaille JC. Ran I, et al. J Neurosci. 2012 May 2;32(18):6335-50. doi: 10.1523/JNEUROSCI.5463-11.2012. J Neurosci. 2012. PMID: 22553039 Free PMC article.
-
A local circuit-basis for spatial navigation and memory processes in hippocampal area CA1.
Geiller T, Priestley JB, Losonczy A. Geiller T, et al. Curr Opin Neurobiol. 2023 Apr;79:102701. doi: 10.1016/j.conb.2023.102701. Epub 2023 Mar 4. Curr Opin Neurobiol. 2023. PMID: 36878147 Free PMC article. Review.
-
From circuit motifs to computations: mapping the behavioral repertoire of cortical interneurons.
Hangya B, Pi HJ, Kvitsiani D, Ranade SP, Kepecs A. Hangya B, et al. Curr Opin Neurobiol. 2014 Jun;26:117-24. doi: 10.1016/j.conb.2014.01.007. Epub 2014 Feb 4. Curr Opin Neurobiol. 2014. PMID: 24508565 Free PMC article. Review.
-
Control of timing, rate and bursts of hippocampal place cells by dendritic and somatic inhibition.
Royer S, Zemelman BV, Losonczy A, Kim J, Chance F, Magee JC, Buzsáki G. Royer S, et al. Nat Neurosci. 2012 Mar 25;15(5):769-75. doi: 10.1038/nn.3077. Nat Neurosci. 2012. PMID: 22446878 Free PMC article.
References
-
- Andersen P, Morris R, Amaral D, Bliss T, O’Keefe J. The Hippocampus Book. New York: Oxford University Press; 2007.
-
- Banks MI, White JA, Pearce RA. Interactions between distinct GABAA circuits in hippocampus. Neuron. 2000;25:449–457. - PubMed
-
- Burgess N, O’Keefe J. Neuronal computations underlying the firing of place cells and their role in navigation. Hippocampus. 1996;6:749–762. - PubMed
-
- Buhl E, Whittington M. Local circuits. In: Andersen P, Morris R, Amaral D, Bliss T, O’Keefe J, editors. The Hippocampus Book. New York: Oxford University Press; 2007. pp. 297–319.
Publication types
MeSH terms
LinkOut - more resources
Full Text Sources
Miscellaneous